Table of Contents
Progress and prospects for energy-efficient applications
Importance of energy efficiency and the role of porous carbon materials
The increasing global focus on sustainability and energy efficiency has a strong influence on developments in materials science, particularly in the field of thermal insulation.
In industrial and commercial applications, efficient thermal insulation is becoming increasingly critical in order to minimize energy losses, reduce operating costs and comply with environmental regulations.
This demand has led to intensive research and development of materials with outstanding insulation properties.
Porous carbon materials, in particular carbon aerogels, are the focus of current research. These materials are characterized by extremely low thermal conductivity which is mainly due to their porous structure and low bulk density.
The unique microstructure of these aerogels – consisting of a network of carbon nanoparticles made up of over 90% air – is the key to their outstanding insulation performance.
Scientific principles of the thermal insulation properties of porous carbon materials
- Porous structure: Carbon aerogels have a structure that is rich in tiny pores.
These pores act as thermal barriers and effectively interrupt the flow of heat through the material.
The high porosity extends the heat flow paths and significantly reduces heat transfer.
Modern measurement techniques such as mercury porosimetry and gas adsorption are used to accurately determine the pore size and distribution, which is crucial for optimizing the insulation properties. - Low bulk density: The low density of carbon aerogels is another decisive factor for their low thermal conductivity.
A lower density here means less solid matter for heat transport and more air-filled spaces that conduct heat poorly.
This makes aerogels excellent insulators that can be used in many industrial applications where traditional materials are too heavy or too inefficient.
Potential applications and benefits in industrial and commercial sectors
- Construction and architecture: In the construction industry, carbon aerogels can be used to improve the thermal insulation of buildings, leading to significant energy savings and improved comfort.
- Aerospace: In the aerospace industry, these lightweight materials can be used to insulate against extreme external temperatures without adding weight.
- Energy storage systems: Carbon aerogels can also be used in energy storage systems to ensure thermal stability and minimize energy losses.
In addition, carbon aerogels are promising candidates for battery applications, especially in supercapacitors, due to their high surface activity and conductivity.
The further development of porous carbon materials could have a significant impact on energy efficiency in a wide range of industries.
Research in this area therefore remains critical to discover new synthesis routes, improvements in material properties and innovative applications.
Fundamentals of thermal conductivity in porous carbon materials
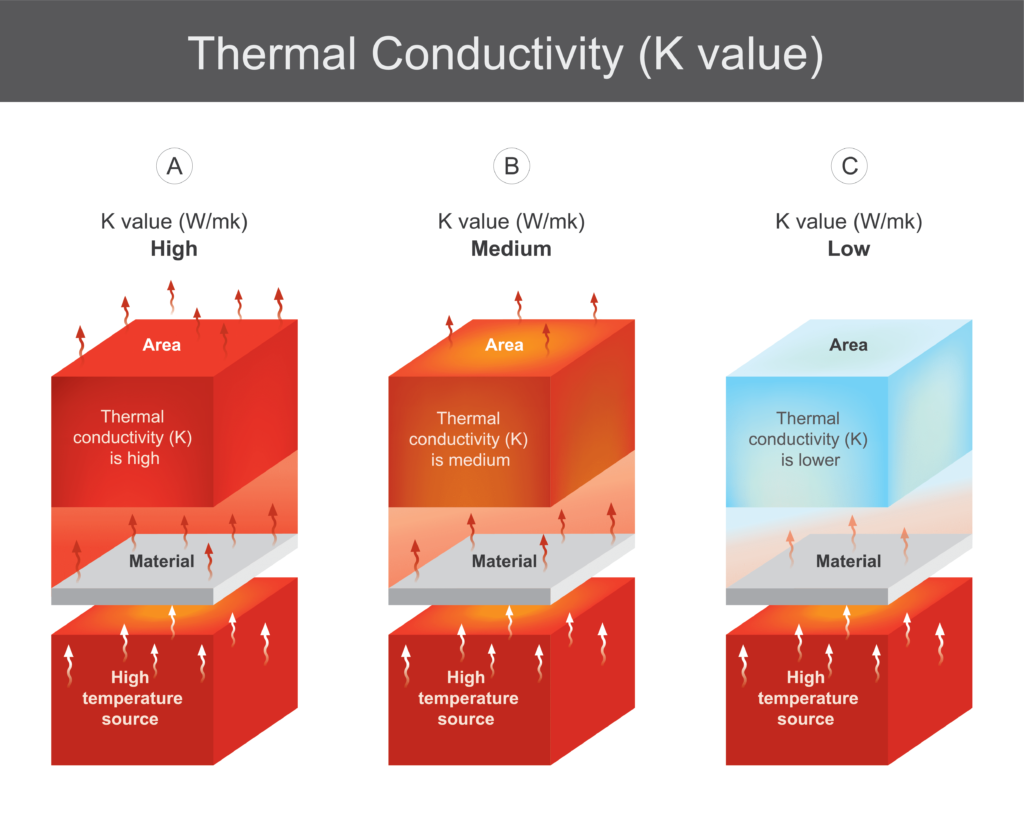
Thermal conductivity is a key physical property that describes how efficiently heat is transported through a material.
In porous carbon materials, this property is influenced by a combination of structural and material factors resulting from the specific characteristics of the carbon and its microstructure:
- Intrinsic thermal conductivity of the solid: Carbon, in its various forms, exhibits high thermal conductivity, which is particularly pronounced in graphite and other graphite-like structures.
In porous structures, such as aerogels, this property is greatly reduced due to the high pore rate and the resulting interruption of the heat conduction paths. - Porosity and bulk density: Porous materials such as carbon aerogels have a high porosity, often over 90%, which results in a very low bulk density.
The many air-filled pores interrupt the path that heat can take through the material, resulting in a significant reduction in effective thermal conductivity.
These pores act as thermal insulators, as the air in the pores has a significantly lower thermal conductivity than the solid carbon. - Thermal conductivity of the gas phase in the pores: In most porous carbon materials, the thermal conductivity of the air in the pores is a decisive factor.
Air has a very low thermal conductivity, which is around 0.024 W/m∙K, compared to pure graphite at room temperature, which has a thermal conductivity of around 120 to 150 W/m∙K This means that the presence of air in the pores significantly reduces the overall thermal conductivity of the material. - Moisture content: Water has a higher thermal conductivity than air (about 0.6 W/m∙K), and its presence in the pores can therefore increase the thermal conductivity of the material.
- Temperature: The thermal conductivity is largely dependent on the temperature.
Graphite typically shows increasing values up to room temperature and then decreasing tendencies.
The porous structure can influence this behavior.
In summary, the thermal conductivity in porous carbon materials is determined by a combination of the material properties of the carbon and the geometric and physical properties of the pore structure.
The control of these factors is crucial for the development and application of these materials in areas ranging from insulation to heat dissipation.
Fundamentals of thermal conductivity in porous carbon materials
Adjusting the thermal conductivity of porous carbon materials is of key importance for many applications, especially when a balance between insulation efficiency and thermal management capability is required.
The following approaches are central to optimizing the thermal properties of these materials:
- Changing the intrinsic thermal conductivity through material modifications:
The intrinsic thermal conductivity of carbon as a base material can be increased through modifications such as graphitization or doping with other elements, which modifies the electron structure and thus the phononic and electronic thermal conductivity of the material.
This offers a targeted adjustment of the thermal properties depending on the desired application. - Adjusting the porosity by changing the manufacturing processes:
By applying pressure during the production of aerogels, for example, the pore volume can be reduced and thus the bulk density increased, resulting in improved thermal conductivity.
Increasing the size of the pores will in turn lead to lower thermal conductivity. - Optimization of the gas phase in the pores:
By filling the pores with gases with a higher thermal conductivity than air, such as helium, the thermal conductivity of the gas phase and therefore the overall thermal conductivity can be increased.
In order to further reduce the thermal conductivity compared to the performance in air, argon, for example, can be used as a pore atmosphere, as argon has a lower thermal conductivity than air.
Another method is to evacuate the pores to completely eliminate the contribution of the gas phase to heat conduction.
This can be useful in applications where extremely low thermal conductivities are required.
These optimization approaches require careful consideration of the material characteristics and the desired thermal performance.
The application of these techniques enables the development of carbon materials that are tailored to specific industrial requirements, from highly efficient thermal insulation to targeted thermal management.
Measurement techniques for determining thermal conductivity
Depending on the application, temperature range and pore size, different techniques can be used to determine the thermal conductivity.
Linseis offers a clearly structured portfolio that is suitable for the characterization of porous carbon materials.
- Transient Hot Bridge (THB): The thermal conductivity can be determined quickly and easily in the temperature range from -150 °C to +700 °C with the available sensors using the method based on the hot-wire method.
- Laser Flash Analyzer (LFA): The universal measuring method of the LFA enables the characterization of porous carbon materials up to extreme temperatures of up to +2800 °C.
It is also possible to vary the ambient conditions and thus investigate the temperature/thermal conductivity as a function of the gas in the pores. - Heat Flow Meter (HFM): For analysis in the lower temperature range of insulation materials, the HFM plate method can also be used, which enables very precise determination of the thermal conductivity up to a maximum of +90 °C.
Effects of porosity on the electrochemical properties
The porosity of carbon materials is not only a key factor for their thermal properties, but also for their function in electrochemical applications.
The large surface area and high porosity enable improved interaction between the active materials and the electrolytes in batteries and supercapacitors:
- Improving ion diffusion: In supercapacitors and batteries, high porosity facilitates the diffusion of ions through the electrode material.
This leads to faster charging and discharging cycles and can improve the power density of the devices. - Increased capacity and energy efficiency: The increased specific surface area provided by high porosity enables a higher activation number of electrolyte ions, which directly improves electrochemical capacity and energy efficiency.
- Optimization of the pore structure: The targeted control of pore size and distribution can further optimize electrochemical performance.
Finely tuned micropores increase surface reactions, while mesopores support mass transport properties, which is particularly important in capacitive desalination and energy storage applications.
In-depth research and optimization of the pore structure will make it possible to further increase the performance of these innovative materials.
Measuring techniques for determining porosity
The precise characterization of the porosity of porous carbon materials is crucial in order to fully understand and optimize their thermal and electrochemical properties.
Porosity directly influences the heat and mass transfer processes within the material.
Various measurement techniques provide detailed insights into the pore structure:
- Nitrogen adsorption (BET method): This technique measures the amount of nitrogen adsorbed on the surface of the material at low temperatures.
The resulting isotherms make it possible to calculate the specific surface area and provide information on the total pore volume and pore size distribution.
For carbon aerogels in particular, the BET method can capture the extremely high surface areas that are responsible for their low thermal conductivity and excellent electrochemical activity. - Electron microscopy: Transmission electron microscopy (TEM) and scanning electron microscopy (SEM) are essential for the direct visualization of micro- and nanostructured pores.
These methods make it possible to observe the morphology and homogeneity of the pore distribution on a very small scale. - Mercury porosimetry: By injecting mercury under varying pressure into the pore network of the material, information about the accessible pore volume and pore size distribution can be obtained.
This method is particularly useful for materials with larger pores and provides insights that other techniques may not capture. - Pycnometry: This method determines the true density of the solid material and compares it with the apparent density of the porous network.
From this, the porosity can be calculated.
Pycnometry is crucial for determining the bulk density of carbon aerogels and other porous structures, which significantly influences the thermal conductivity.
Conclusions and outlook
Porous carbon materials such as carbon aerogels represent an outstanding advance in materials science, particularly due to their excellent thermal and electrochemical properties.
These materials not only offer potential for improved energy efficiency in a variety of applications, but also for the development of new technologies that are characterized by environmental protection and sustainability.
Expanding the areas of application: In addition to their use in thermal insulation and electrochemical applications such as batteries and supercapacitors, porous carbon materials could also find new areas of use in medical technology, water purification and sensor technology. Their porous structure could, for example, be used to develop highly sensitive biosensors or efficient filter systems to remove pollutants or pathogens from water and air.
Further development of material synthesis: The synthesis of porous carbon materials is a crucial field of research that is constantly being developed. New synthesis processes that are more cost-efficient and use more environmentally friendly starting materials could significantly increase the spread and application of these materials. Innovative approaches such as the use of biomass as a carbon source or the development of environmentally friendly solvents and catalysts to control pore formation could reduce production costs and minimize environmental impact.
Characterization and tailor-made material designs: The continuous improvement of analytical techniques will make it possible to determine and optimize the properties of porous carbon materials even more precisely. Advances in microscopy and spectroscopy can help to understand the pore structure and chemical composition at the molecular level. This knowledge can be used to develop materials with tailored pore sizes, specific surface areas and specifically adjusted thermal conductivities in order to optimize them for specific industrial applications.
Porous carbon materials represent a promising solution for increasing energy efficiency in numerous application areas.
Ongoing research and development in this area is expected to further improve the properties of these materials, which could make them a key to future energy-saving measures.