Description
To the point
Quenching in metallurgy is the rapid cooling of a heated metal in a quenching medium such as water, oil or air to achieve the desired material properties. In metallurgy, quenching is one of the critical steps in the heat treatment of a metal and is normally used to harden the final steel product.
There are three main types of transformation diagrams that are useful in selecting the optimum steel and processing route to achieve a particular range of properties.
These are time-temperature transformation diagrams (TTT), continuous cooling transformation diagrams (CCT) and continuous heating transformation diagrams (CHT).
With the high-speed dilatometer with induction furnace, you can create TTT, CHT and CCT diagrams in a temperature range from -150 °C to 1600 °C (various models) according to ASTM A1033 – 04 create.
Deformation of metals: If a sufficient load is applied to a metal or other structural material, this leads to a change in the shape of the material. This change in shape is called deformation.
It is caused either by the mechanical effect of external forces or by various physical and physico-chemical processes. Deformed or mechanically processed metals are far superior to the cast metals from which they are made.
Stress-strain diagrams are a very important graphical measure of the mechanical properties of a material.
The diagram provides information on many mechanical properties such as strength, toughness, elasticity, yield strength, strain energy, elasticity and elongation under load.
The stress-strain diagram expresses a relationship between a force exerted on a material and the deformation of the material caused by this force.
The stress-strain diagram is determined by tensile tests.
Tensile tests are carried out in tensile testing machines (DIL L78 Q/D/T) in which a controlled, uniformly increasing tensile force is applied to the specimen.
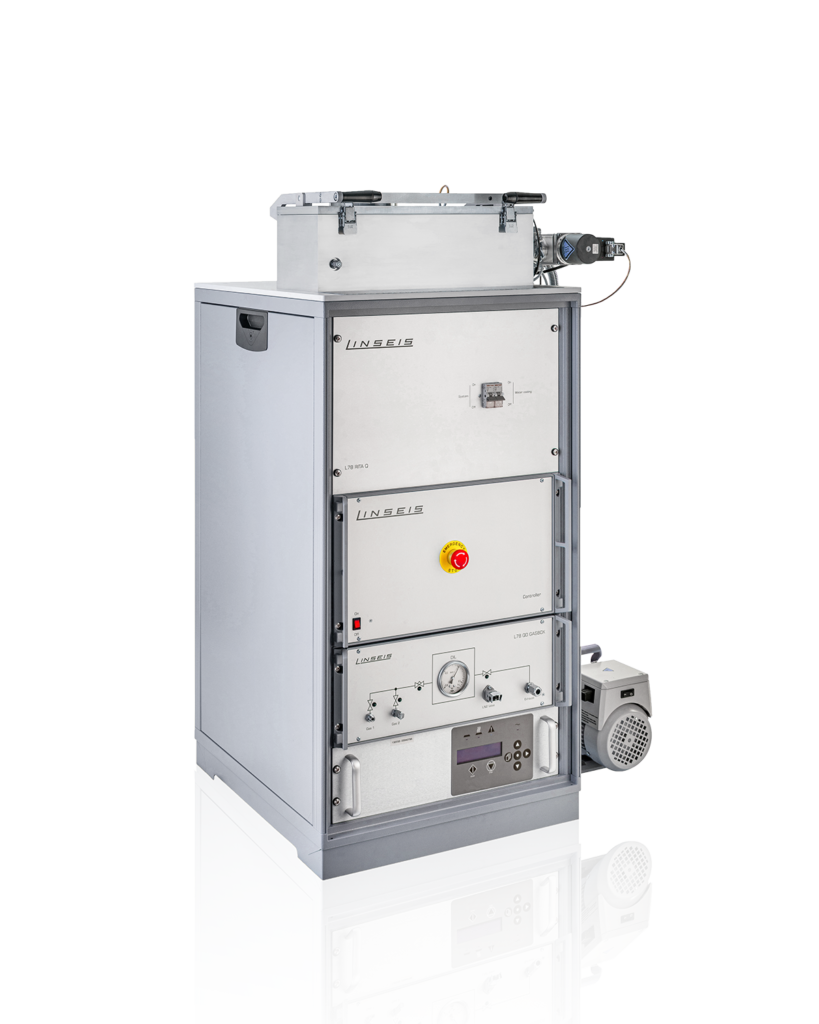
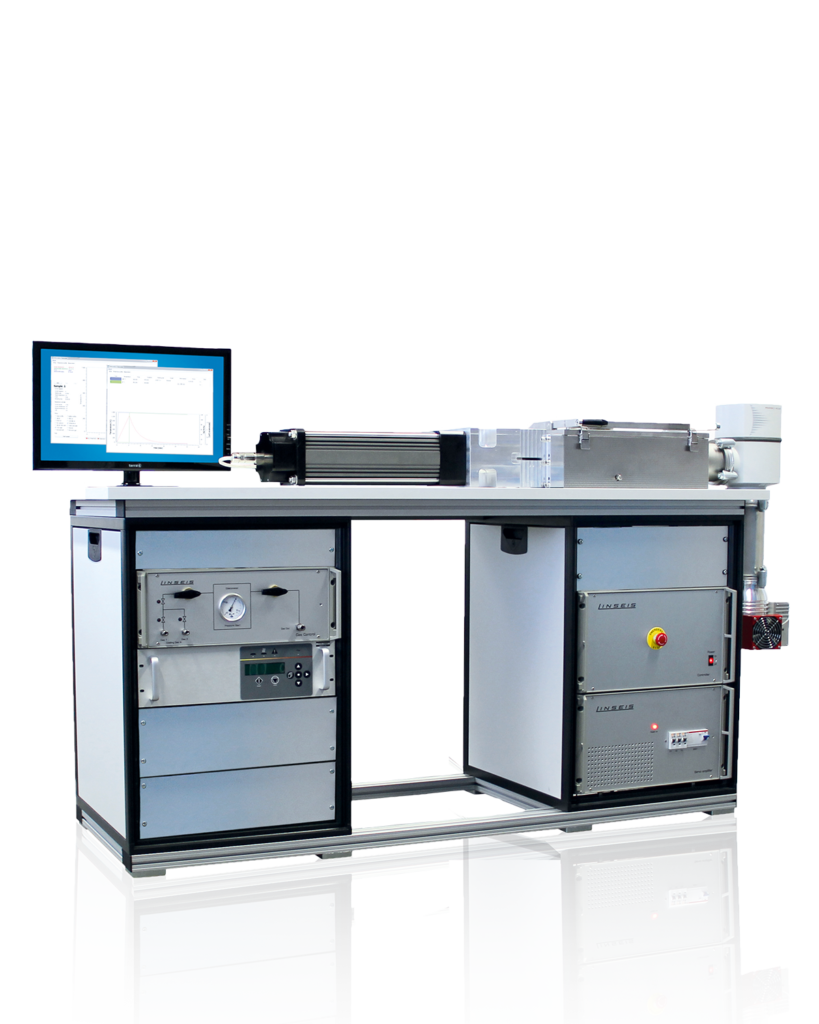
The advantages of the L78 RITA quenching dilatometer:
- The device can perform measurements under vacuum, inert, oxidizing, reducing atmospheres from -150 °C (low temperature option) to 1600 °C in one run.
- The unique heating and cooling arrangement enables very fast controlled heating and cooling speeds of up to
4000 K / s. - Non-metallic samples can be analyzed with the optional susceptor.
- This special quenching dilatometer is specially designed for the determination of continuous cooling/heating CHT, CCT and isothermal TTT diagrams.
Unique features
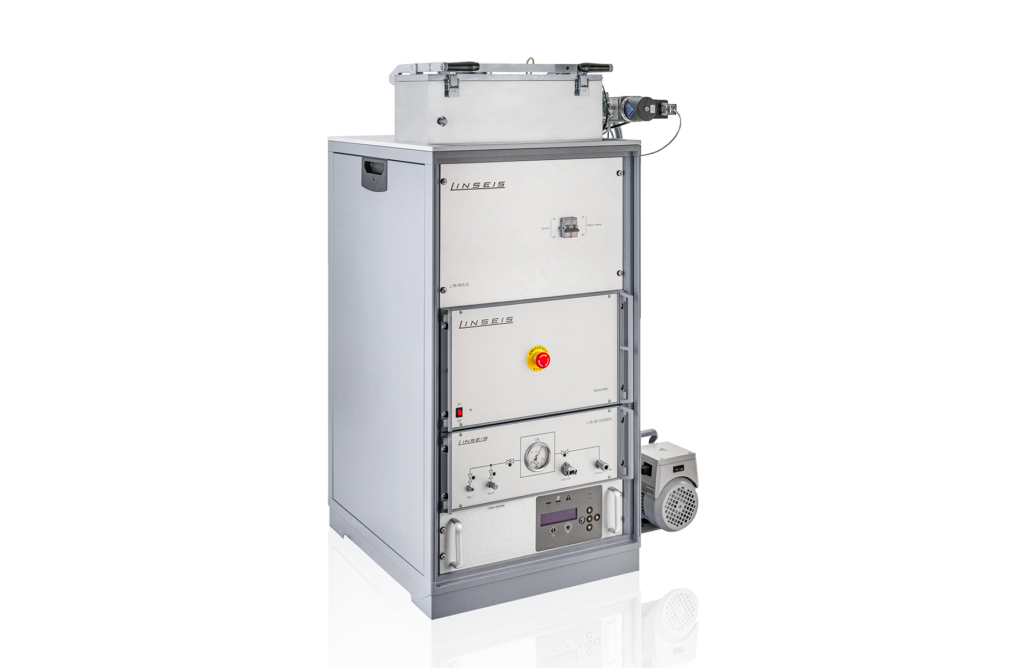
Quick quenching with water, oil or air to improve hardness
Determination of TTT, CHT and CCT diagrams
in the temperature range from -150°C to 1600°C
Heating and cooling rates
to 2500°C/s
Use of induction furnaces and
high-speed dilatometers
for precise measurements
Questions? We're just a call away!
+01 (609) 223 2040
+49 (0) 9287/880 0
Our service is available Monday to
Thursday from 8 am to 4 pm
and Friday from 8 am to 12 pm.
We are here for you!
Specifications
MODELL | DIL L78/RITA Q * |
---|---|
Ofen: | Induktionsofen |
Temperaturbereich: | RT up to 1600°C (-150°C to 1600°C mit Kryo-Option) |
Probenbeschaffenheit: | feste / hohle Proben |
Probendurchmesser: | 3mm oder 4mm (reduzierte Heiz- und Kühlrate) oder hohler AD 4mm ID 3 bis 3,5 mm |
ProbenLänge: | 10 mm |
Heizraten: | ≤ 4000 K / s |
Kühlraten: | ≤ 4000 K / s |
Messung von Längenänderungen: | +/- 1.2mm |
*Spezifikationen hängen von den Konfigurationen ab |
MODELL | DIL L78/RITA Q/D * |
---|---|
Ofen: | Induktionsofen |
Temperaturbereich: | -100°C bis 1600°C |
Probenbeschaffenheit: | feste / hohle Proben |
Probendurchmesser: | ø 3 mm |
Probenlänge: | 10 mm |
Heizraten: | ≤ 4000 K / s |
Kühlraten: | ≤ 2500 K / s |
Messung der Längenänderung | +/- 1,2 mm (0,01 µm Auflösung) |
Abtastrate (für Temperatur, Länge, Kraft): | ≤ 1 kHz |
*Spezifikationen hängen von den Konfigurationen ab |
MODELL | DIL L78/RITA Q/D/T* |
---|---|
Ofen: | Induktionsofen |
Temperaturbereich: | RT up to 1600°C |
Probenbeschaffenheit: | feste Proben |
Probendurchmesser: | ø 5 mm |
Probenlänge: | 10 mm |
Heizraten: | ≤ 125 K / s |
Kühlraten: | ≤ 125 K / s |
Heiz- und Kühlraten (Kombinierte Umformung): | max. 100 K / s |
Umformkraft | 22 kN |
Umformgeschwindigkeit: | 0,01 bis 100 mm / s (mehr auf Anfrage) |
Umformgrad: | 0,02 bis 1,2 ms |
Messung der Längenänderung: | +/- 5 mm (Auflösung 0,05 um) |
Abtastrate (für Temperatur, Länge, Kraft): | ≤ 1 kHz |
Mindestpause zwischen zwei Verformungsschritten: | 60 ms |
Atmosphäre: | Schutzgase, Vakuum bis zu 10E-5 mbar |
Mechanische Steuerungs-Modi: | Hub, Kraft, Dehnrate (optional) |
*Spezifikationen hängen von den Konfigurationen ab |
Accessories
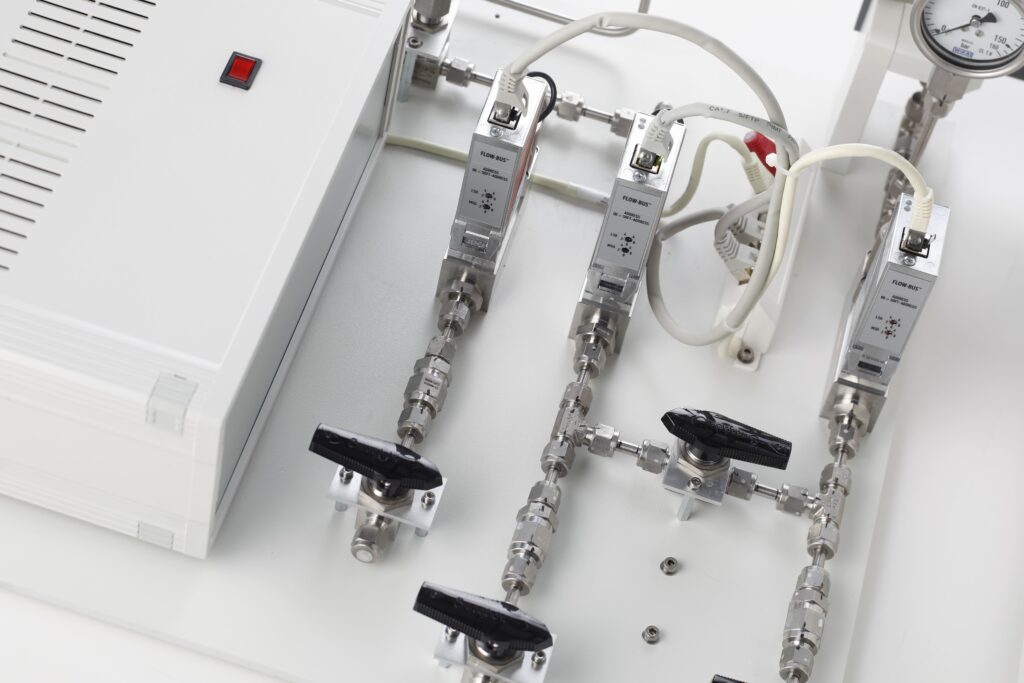
- Devices for sample preparation
- Caliper for manual or online entry of the sample length
- Various gas boxes: manual, semi-automatic and MFC controlled Various rotary and turbomolecular pumps
- Thermocouple welder
- Low temperature option (LN2 cooling)
Software
Making values visible and comparable
- Glass transition and softening point determination
- Automatic softening point switch-off, freely adjustable (system protection)
- Display of absolute or relative shrinkage or expansion
- Representation and calculation of technical / physical expansion coefficients
- Rate-controlled sintering (software option)
- Sintering process evaluation
- Density determination
- Automatic evaluation routines
- System correction (temperature, zero curve, etc.)
- Automatic zero point adjustment
- Automatic punch contact pressure control
- Real-time color display
- Automatic and manual scaling
- Display of the axes freely selectable (e.g. temperature e.g. temperature (x-axis) against delta L (y-axis))
- Mathematical calculations (e.g. first and second derivatives)
- Saving complete evaluations
- Multitasking function
- Multi-user function
- Zoom option for various curve sections
- Any number of curves can be loaded on top of each other for comparison
- Online Help Menu
- Free labeling
- EXCEL® and ASCII export of measurement data
- Data smoothing
- Zero curves are offset
- Cursor function
- Statistical curve evaluation (mean value curve with confidence interval)
- Tabular printout of the data and expansion coefficients
- Calculation of Alpha Phys, Alpha Tech, relative expansion L/L0
- Curve arithmetic, addition, subtraction, multiplication
Applications
Phase transformation of steel
The L78 Q and L78 Q/D dilatometers can be used to measure phase transformations in steel very accurately up to high heating and cooling rates.
The transitions between different steel phases and the temperatures at which they occur are crucial for the creation of the TTT, CCT and CHT diagrams.
In this example, the steel sample is heated above its austenitic temperature in an initial ramp.
The sample is then quenched and cooled.
The diagram shows the beginning (Ar3) and the end (Ar1) of the phase transformation from austenite to ferrite.
These two temperature points can then be fitted to a CCT diagram based on the quenching rate.
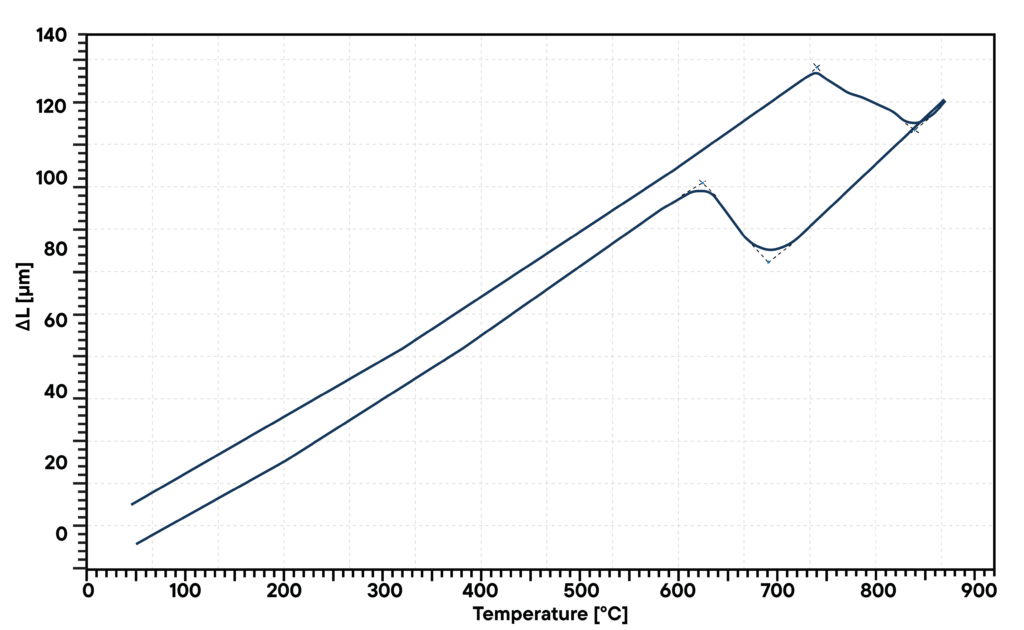
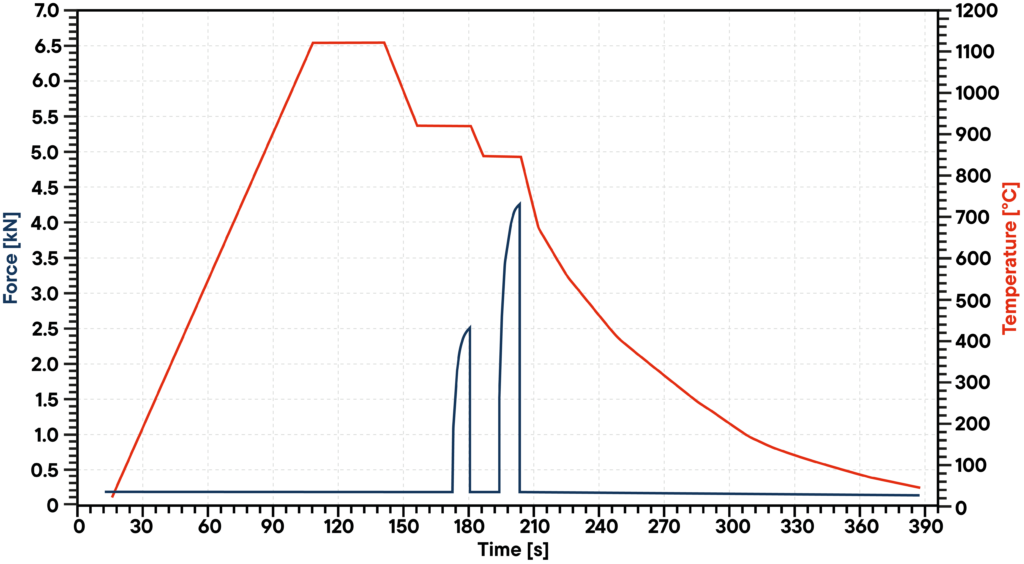
2-Step Deformation Test
The L78 Q/D is the ideal instrument for optimizing the quenching rate after multi-stage deformations. These measurements can be used to simulate the processing of steel in order to control the crystalline structure and physical properties.
In this example, after the initial heating and resulting thermal expansion, the steel package is kept isothermal and undergoes a series of two deformation steps: a first deformation of 1 mm over a period of 10 s, followed by a second deformation of 1 mm over a further period of 10 s. After the deformation steps, the material is quenched and contraction and phase transformation are measured. After the deformation steps, the material is quenched and the contraction and phase transformation measured. This data can be used by manufacturers to optimize their production processes for steels with the desired physical properties.
Videos
Well informed