Description
To the point
Information about thermophysical properties of materials and heat
transfer optimization of final products is becoming more and more vital
for industrial applications. Over the past few decades, non-distructive
optical methods have developed into the most commonly used technique for the measurement of the thermal diffusivity and thermal conductivity of various kinds of solids, powders and liquids.
Thermophysical properties of thin-films are becoming more and more important in industries such as, phase-change optical disk media, thermo-electric materials, light emitting diodes (LEDs), phase change memories, flat panel displays, and the semiconductor industry.
All these industries deposit a film on a substrate in order to give a device a particular function. Since the physical properties of these films differ from those of bulk materials, these data are required for accurate thermal management predictions.
Thermal properties:
- Thermal Conductivity
- Volumetric Heat Capacity
- Thermal Diffusivity
- Thermal Efficiency
- Thermal Boundary Conductance
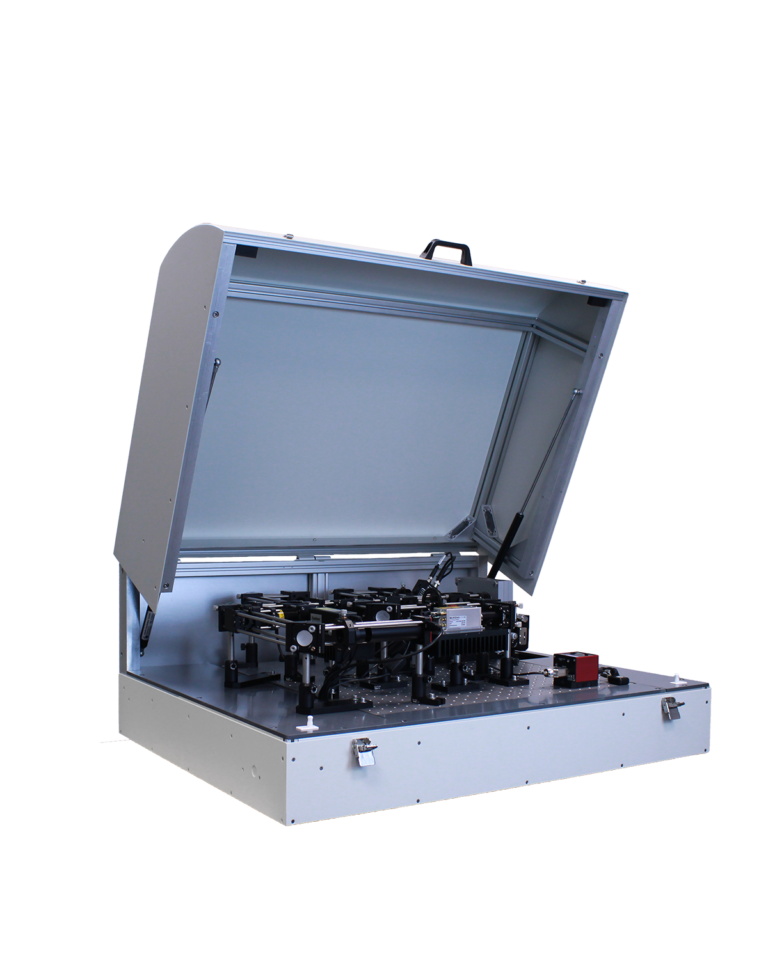
Thin films:
Thin films are materials with thicknesses from nanometers to micrometers, applied to surfaces.
Their thermophysical properties differ significantly from those of bulk materials depending on thickness and temperature. Thin films are typically used in semiconductors, LEDs, fuel cells, and optical storage media.
Different types of thin films
- Thin film: layer of a few nm to µm
- Films are grown on specific substrate
- Typical growing techniques include
- PVD (e.g. sputtering, thermal vaporization)
- CVD (PECVD, LPCVD, ALD)
- Drop casting, Spin coating & Printing
- Various kinds of films, including:
- Semiconducting films (e.g. thermoelectric, sensors, transistors)
- Metallic films (used as contacts)
- Thermal barrier coatings
- Optical coatings
Multilayer sample
Thin film
(e.g. semiconductor, metal, organic, oxide)
Substrates
(e.g. Si, Si3N4, Fused Silicia)
FDTR
Frequency Domain
FDTR is a contactless characterization technique for thin film thermal material properties in the frequency domain, measuring the thermal properties of thin films. This method utilizes the effect of thermoreflectance to establish a highly sensitive thermometer that detects the surface temperature of the sample by monitoring its reflectivity.
A continuous wave laser (Probe laser) with 532 nm wavelength is used for detection, while heating is achieved with a harmonically modulated pump laser at a different wavelength (405 nm). Local heating induces changes in the reflectivity, and the phase lag between the thermal excitation and the detection is measured using a lock-in amplifier.
Modelling the response in the frequency domain with a diffusive heat transport model allows us to determine the thermal conductivity, volumetric heat capacity, thermal diffusivity, thermal effusivity and thermal interface conductance.
A thin metallic transducer layer (60 -70 nm in thickness) is deposited on top of the surface of the samples to enhance the temperature coefficient of reflectance, dR/dT, and at the same time to reduce the optical penetration depth in the material.
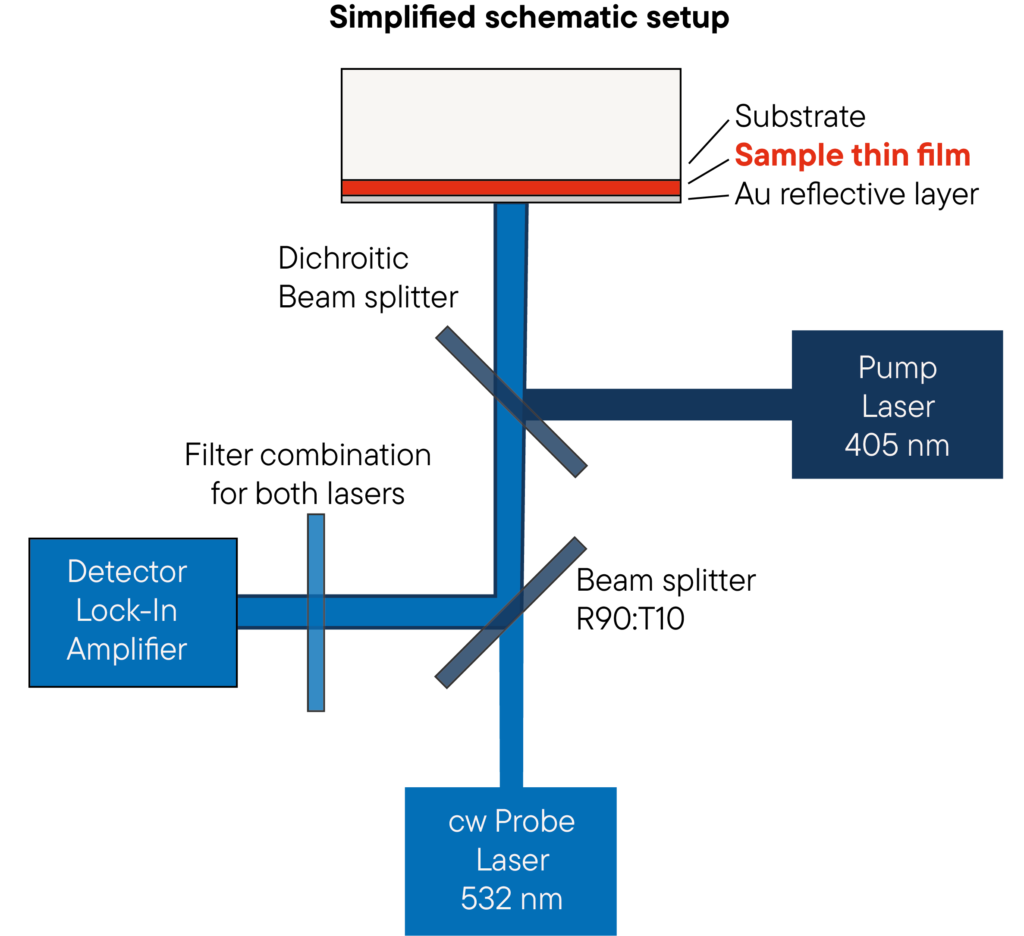
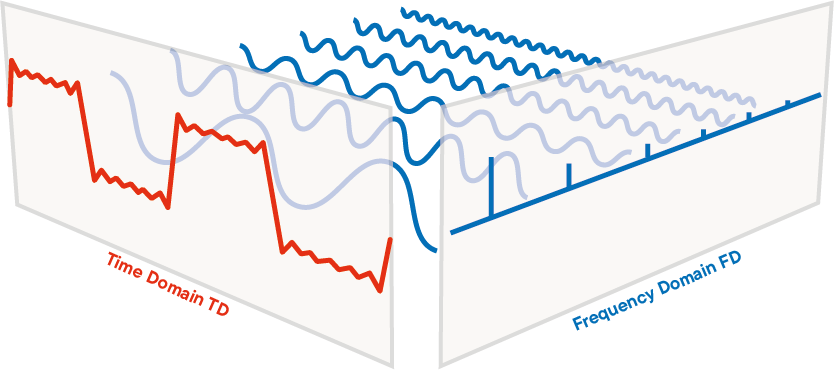
Advantages:
- Broader measuring range
- Easier handling
- Higher stability
- More precise results
- Ability to measure thermal contact resistance between two
layers - No need for assumptions about
heat capacity and density of thin
sample films
Comparison of FDTR and TDTR methods
Our advanced FDTR (Frequency-Domain Thermoreflectance) system offers significant advantages over the traditional TDTR (Time-Domain Thermoreflectance) method, optimizing the setup and enhancing measurement stability.
No need for probe laser adjustment: Unlike the TDTR arrangement, where the probe laser must be adjusted relative to the sample due to slight changes in reflection when the sample is altered, our FDTR system eliminates this requirement. Our system includes automatic focusing, which continuously adjusts the probe laser‘s focus to accommodate any changes in the sample, ensuring optimal measurement conditions without manual intervention
Aligned lasers: With perfectly aligned lasers in our FDTR system, there is no need to adjust the probe laser beam, resulting in a simpler sample setup and more stable measurements.
Broader measuring range: Our FDTR outperforms even nano-pulsed TDTR setups with a broader measuring range. Thinner samples layers and thin films with higher thermal conductivity can be measured.
No need for assumptions: Our comprehensive evaluation algorithm allows you to measure thin films without any assumptions. All you need to know is the sample thickness
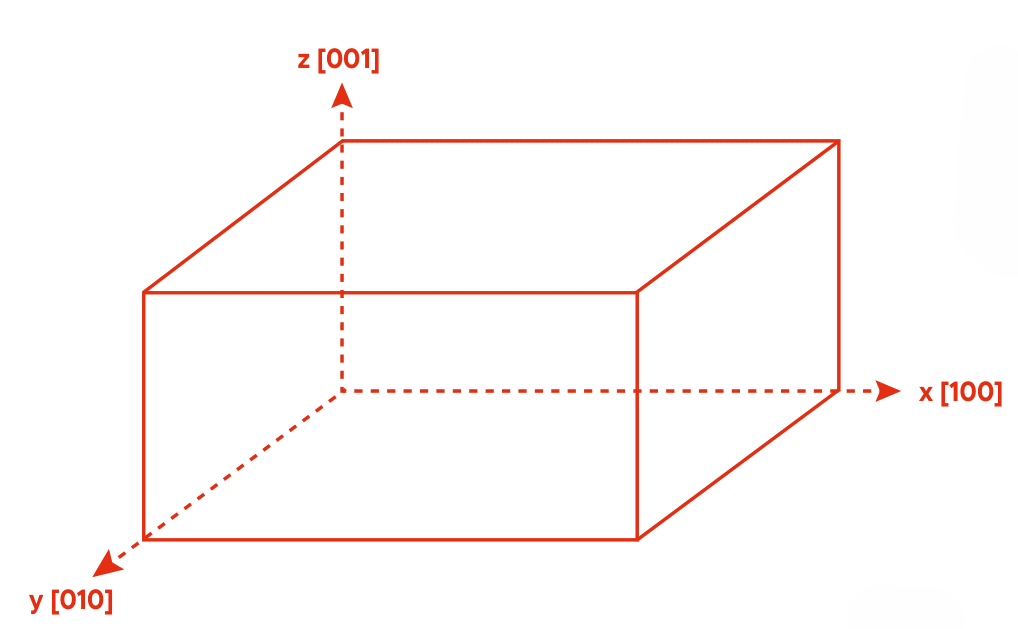
Thermal conductivity anisotrophy
In the development of new batteries, the flow direction of the emerging heat
during operation plays a crucial role. Therefore, it is important to know, that the thermal conductivity can differ in different directions within the material, which is called anisotrophy. This typically occurs in thin films. The two main axes have special names: one is perpendicular to the surface, known as cross-plane, while in-plane refers to heat flow parallel to the surface.
Understanding both types is essential, especially for materials used in electronics, where efficient heat dissipation is critical.
In-plane thermal conductivity is particularly important in battery materials to
manage heat flow across cell layers, impacting safety and efficiency. On the other hand, thermal barrier coatings rely on low cross-plane thermal conductivity making them ideal for applications where heat should be isolated to protect sensitive underlying components. An example for that kind of coating material is silicon dioxide SiO₂ thin films.
Two-dimensional materials, like PdSe crystals, offer highly exiting chances in the evolution of efficient energy conversion and thermal management, due to the anisotropic structure and so thermal properties. To entangle
these features and exploit these unique attributes, anisotropic thermal investigations have to be done.
Figure 2: Out-of-plane and in-plane thermal conductivity of a 297 nm thick PdSe2.
a) Out-of-plane thermal conductivity of PdSe2 along
crystallographic plane [001].*
b) In-plane thermal conductivity of PdSe2 along crystallographic plane [100].*
c) In-plane thermal conductivity of PdSe2 along crystallographic plane [010].*
The TF-LFA offers the possibility to measure the thermal conductivity of such a 2D Material not only in both
major directions, in- and out-of-plane, (see Fig. 2 b & 2 c) even over the axis of rotation of the surface in two
different crystallographic planes.
*Measurements were provided by Dr. Juan Sebastian Reparaz
Unique features
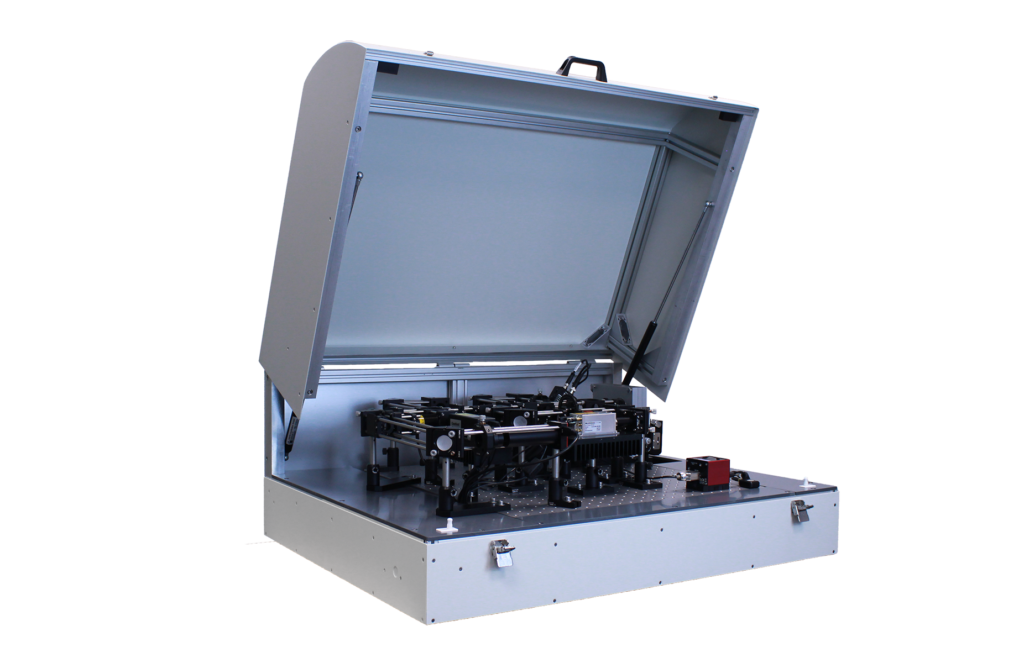
Comprehensive thermal characterization:
- Measurement of thermal conductivity,
heat capacity, thermal diffusivity and
thermal effusivity - Determination of the thermal contact
between two neighboring layers
Anisotropy function:
- Optional function for measuring the thermal
conductivity both in the direction of passage
(through the material) and in the plane
(perpendicular to the laser excitation)
Wide temperature range:
- The device can measure the thermal properties
of thin films at room temperature up to 500°C
Thermal imaging:
- With the optional sample
mapping function, the thermal
properties of the sample can be
tracked over a specific area or
points of the surface, ideal for
homogeneity tests
Automatic optimization and camera option:
- Automatic optimization of the laser beam
to improve the measurement results - Additional camera option that provides
visual information and facilitates the selection
of interesting spots on the sample surface
Measurement of thermal contact
resistances/conductive values:
- Measurement of the thermal
contact between two layers,
e.g. between sample and surface
or sample and transducer layer
Questions? We're just a call away!
+1 (609) 223 2070
+49 (0) 9287/880 0
Our service is available Monday to
Thursday from 8 am to 4 pm
and Friday from 8 am to 12 pm.
We are here for you!
Specifications
Hard Facts
MODEL | TF-LFA |
---|---|
Sample dimensions: | Any shape between 2mm x 2mm and 25mm x 25mm lateral size |
Thin film samples: | 10nm up to 20μm* (depends on sample) |
Temperature range: | RT, RT up to 200/500°C Sample holder for 4“ Wafer (only RT) |
Measured properties: | Thermal conductivity Thermal diffusivity Thermal interface resistance Volumetric specific heat capacitiy Thermal effusivity |
Options: | Anisotrophy: Measurement of cross-plane and in-plane thermal properties Sample mapping: Scanning multiple positions of the sample pointwise or clusterwise. Mapping area: 10 mm² Stepsize: 50 μm Camera: Allows the user to view the present sample surface and the position of the laser beams to record the actual measurement position. |
Atmosphere: | inert, oxidizing or reducing vaccuum up to 10E-4mbar |
Diffusivity measuring range: | 0.01mm2/s up to 1200mm2/s (depends on sample) |
Pump laser: | CW Laser (405 nm, 300 mW, modulations frequency up to 200 MHz) |
Probe laser: | CW Laser (532 nm, 25 mW) |
Photodetector: | Si Avalanche Photodetector, active diameter: 0.2 mm, bandwidth: DC - 400MHz |
Power supply: | AC 100V ~ 240V, 50/60 Hz, 1 kVA |
Software: | Included. Software package using multi-layer analysis for calculation of thermophysical properties |
*Actual thickness range depends on sample |
Data sheet
Software
Making values visible and comparable
All LINSEIS thermoanalytical devices are PC-controlled, the individual software modules run exclusively under Microsoft® Windows® operating systems.
The complete software consists of 3 modules: temperature control, data acquisition and data evaluation.
As with other thermoanalytical experiments, the LINSEIS software offers all the essential functions for preparing, carrying out and evaluating the measurements.
Thanks to our specialists and application experts, LINSEIS has succeeded in developing this easy-to-understand and highly practical software.
General software
- Fully compatible with MS® Windows™
- Data security in the event of a power failure
- Evaluation of the current measurement
- Comparison of the curves
- Storage and export of evaluations
- Export and import of ASCII data
- Data export to MS Excel
Evaluation software
- Determination of contact resistance
- Multilayer heat transport model
to extract the thermal conductivity, thermal diffusivity, thermal
effusivity and volumetric heat capacity at once - Measurement feasibility check
- Sensitivity Plot
Measuring software
- Easy and user-friendly data input for temperture segments, gases etc.
- Fully automated measurement
Applications
Application example: SiO2 thin film 504 nm
Thin film glass layers out of pure silicon dioxide (Quartz) are frequently used in semiconductor and electronic industries as protective layer or thermo or electronical insulation layers. In this example SiO2 layer was investigated using the Linseis TF-LFA device to fully characterize its thermal properties.
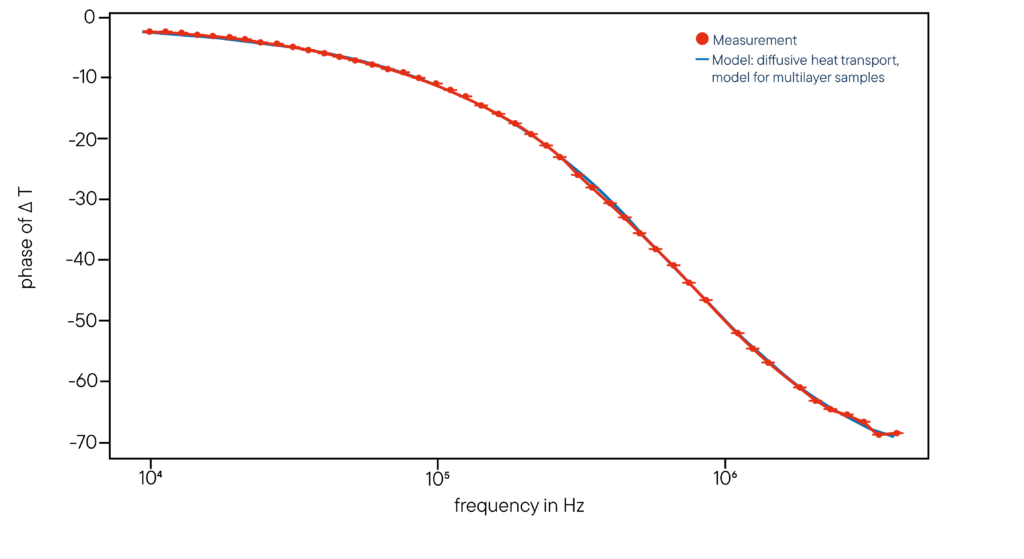
Aluminum nitride AIN 200 nm
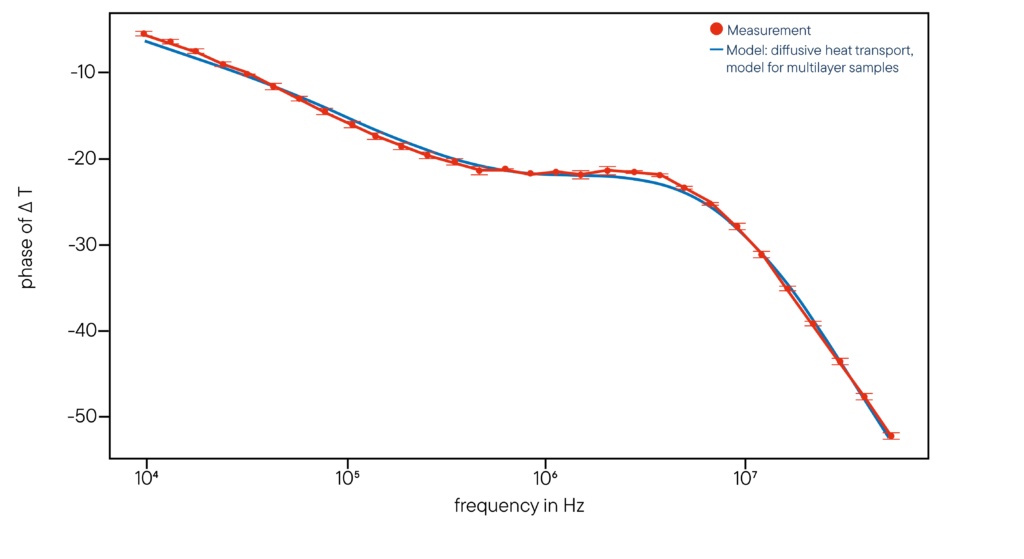
Application example: Aluminum nitride AIN
AlN is frequently used as thermal insulation layer or electronical insulation layer in sensors or microelectronics. Its thermal properties depending on layer thickness were investigated by TF-LFA in this application.
Aluminum nitride AIN 800 nm
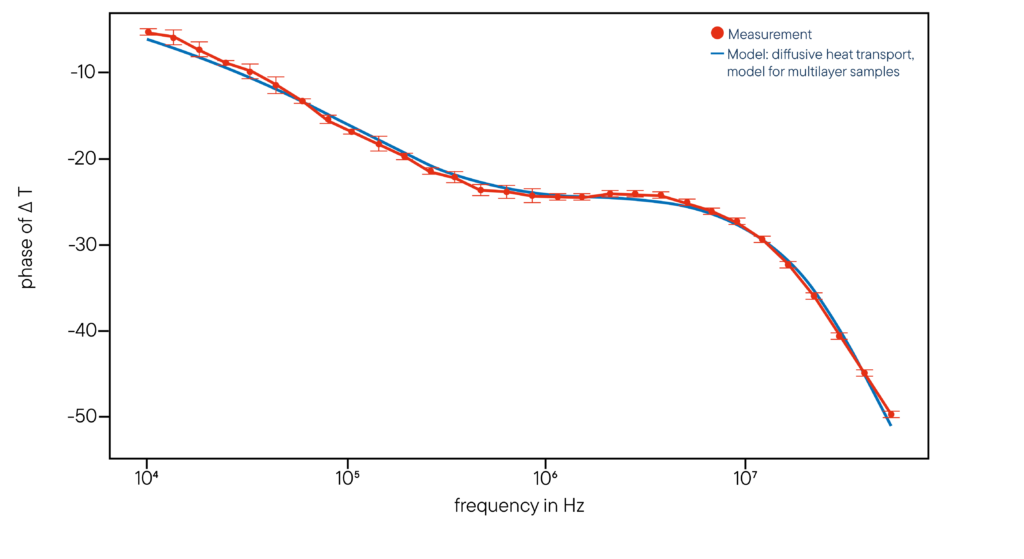
Aluminum nitride AIN 1600 nm
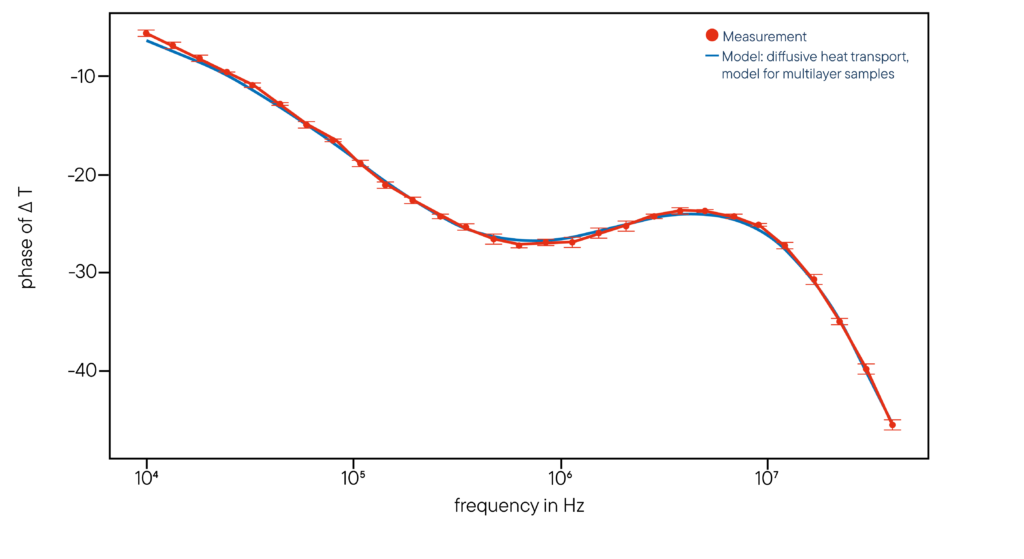
Application example: CVD Diamond – Thermal Conductivity
High-conductivity diamond samples can be measured using the Linseis Laser Frequency Analyzer (TF-LFA), which utilizes the Frequency Domain Thermoreflectance technique to characterize thermal behavior and
ensure quality control in applications where efficient heat dissipation is critical. Accurate thermal conductivity measurements are essential to verify the quality and performance of diamond samples, as factors such as grain size, purity, and thickness can influence the transport properties.
Measurement of the thermal properties of CVD diamond. The x-axis displays the logarithmically scaled frequency in Hertz, while the y-axis represents the phase shift between the excitation by the pump laser and the probe laser. Where λ is the thermal conductivity, α is the thermal diffusivity, e is the thermal effusivity and TBC is the thermal boundary conductance between the transducer layer (gold) and the sample (diamond). It determines how well a combination of materials is able to exchange heat with each other.
Frequency Domain Thermoreflectance (FDTR) is a preferred method for measuring thermal conductivity in materials like CVD diamond, especially in thin films and micro-scale samples where high spatial resolution is
essential. The Linseis Laser Frequency Analyzer (TF-LFA) is an ideal tool for this purpose. FDTR uses a modulated laser to induce localized heating in the sample and measures the material‘s thermoreflectance response
at varying modulation frequencies. This technique allows researchers to determine thermal conductivity by modeling the heat flow through the diamond and its interfaces.
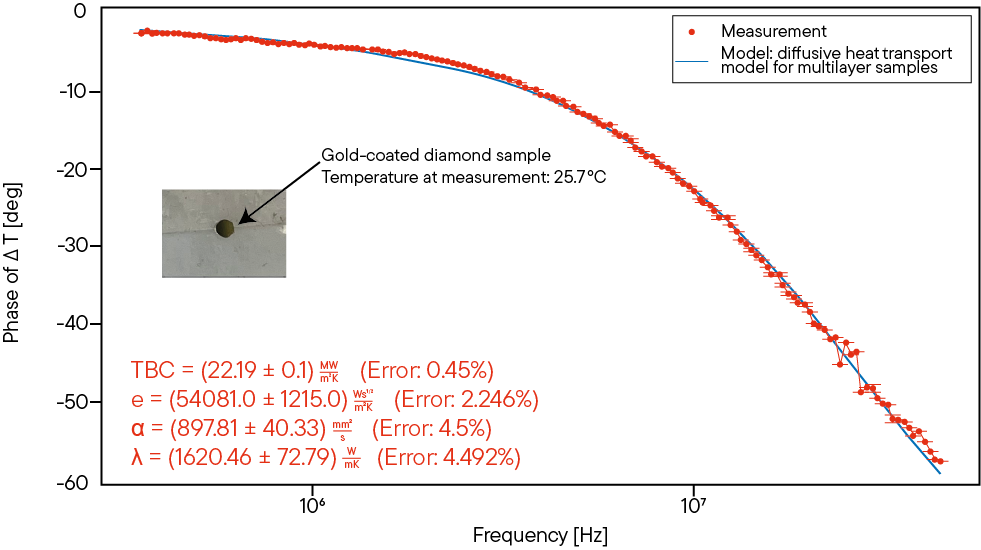
Well informed