Diamonds are known for their exceptional thermal conductivity known. CVD (Chemical Vapor Deposition) diamond samples usually achieve values between 1000 and 2200 W/mK [1,2]rare and high-purity samples even up to 3320 W/mK. [2,3] This property makes diamond an ideal candidate for heat dissipation in high-performance electronics, laser systems and other applications that require efficient thermal management. [ 4] Accurate measurement of the thermal conductivity of diamond samples is crucial for optimizing material quality and understanding its performance in demanding thermal environments.
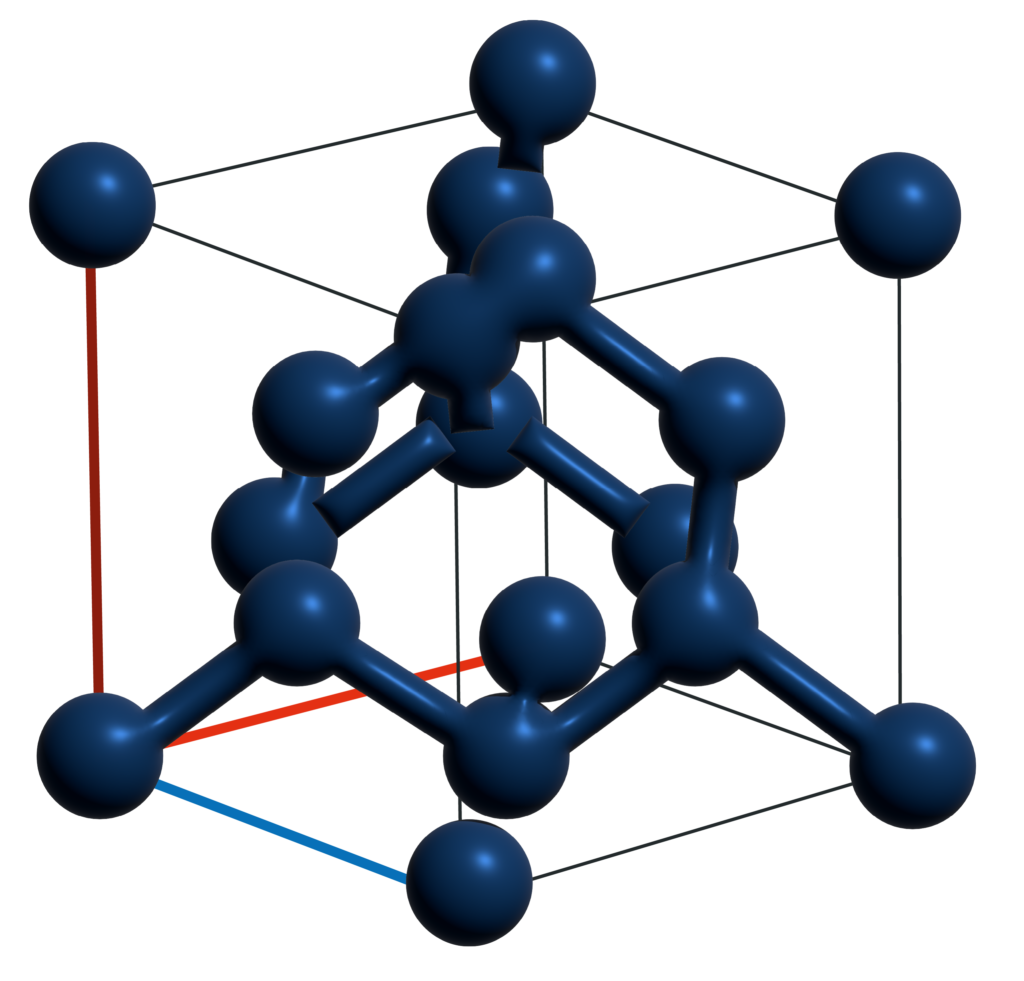
Why do diamonds have such high thermal conductivity and thermal diffusivity?
The thermal conductivity of diamonds results from their unique atomic structure and properties [2,3]:
1. Strong Covalent Bonds: The three-dimensional tetrahedral structure, in which each carbon atom is covalently bonded to four others, creates a rigid lattice that efficiently transfers heat.
2. Low Atomic Mass: carbon atoms are relatively light, so they can vibrate quickly, which facilitates the rapid transfer of heat through lattice vibrations, also known as phonons, which transport heat quickly.
3. High Phonon Velocity: High phonon speed due to its rigidity and strong interatomic forces. This allows thermal energy to travel faster through the lattice.
4. High Debye temperature: The structure of the diamond supports high-frequency vibrations, even at high temperatures, and thus maintains thermal conductivity. [4]
5. Low Phonon Scattering: The symmetrical crystal structure minimizes scattering so that phonons can travel long distances without losing energy. [4]
6 Isotopic Purity: The uniform atomic mass of a diamond further reduces scattering and improves phonon propagation. [6]
These factors make diamonds ideal for applications that require high thermal conductivity, such as the cooling of electronics and high-power laser systems.
Diamond samples with high conductivity can be analyzed with the Linseis Laser Frequency Analyzer (TF-LFA) which uses the Frequency Domain Thermoreflectance technique to characterize thermal behavior and ensure quality control in applications where efficient heat dissipation is critical. Accurate thermal conductivity measurements are essential to verify the quality and performance of diamond samples, as factors such as grain size, purity and thickness can affect transport properties.
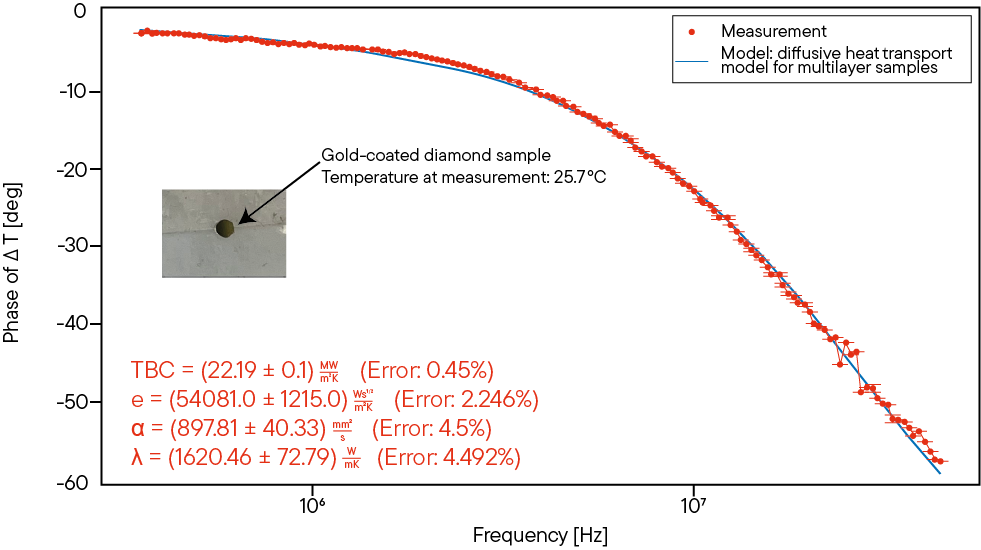
Frequency domain thermoreflectance (FDTR) is a preferred method for measuring thermal conductivity in materials such as CVD diamond, especially in thin films and micro-scale samples where high spatial resolution is essential. The Linseis Laser Frequency Analyzer (TF-LFA) is an ideal tool for this purpose. FDTR uses a modulated laser to induce localized heating in the sample and measures the thermoreflectance response of the material at different modulation frequencies. This technique allows researchers to determine the thermal conductivity by modeling the heat flow through the diamond and its interfaces.
Sources:
[1] M. Shamsa, S. Ghosh, I. Calizo, V. Ralchenko, A. Popovich, A. A. Balandin; Thermal conductivity of nitrogenated ultrananocrystalline diamond films on silicon. J. Appl. Phys. April 15, 2008; 103 (8): 083538. https://doi.org/10.1063/1.2907865
[2] Zhang, Chunyan & Vispute, Ratnakar & Fu, Kelvin & Ni, Chaoying. (2023). A review of thermal properties of CVD diamond films. Journal of Materials Science. 58. 1-23 . https://doi.org/10.1007/s10853-023-08232-w.
[3] Wei L, Kuo PK, Thomas RL, Anthony TR, Banholzer WF (1993) Thermal conductivity of isotopically modified single crystal diamond. Phys Rev Lett 70(24):3764-3767. https://doi.org/10.1103/PhysRevLett.70.3764
[4] Pop E, Varshney V, Roy AK (2012) Thermal properties of graphene: fundamentals and applications. MRS Bull 37(12):1273-1281. https://doi.org/10.1557/mrs.2012.203
[5] Mashali F, Languri E, Mirshekari G, Davidson J, Kerns D (2019) Nanodiamond nanofluid microstructural and thermo-electrical characterization. Int Commun Heat Mass Transfer 101:82-88. https://doi.org/10.1016/j.icheatmasstransfer.2019.01.007
[6] Angadi MA, Watanabe T, Bodapati A, Xiao X, Auciello O, Carlisle JA, Eastman JA, Keblinski P, Schelling PK, Phillpot SR (2006) Thermal transport and grain boundary conductance in ultrananocrystalline diamond thin films. J Appl Phys. https://doi.org/10.1063/1.2199974.