Table of Contents
Applications of Molten Salts
Molten salts are stable at high temperatures above 300 °C and exhibit remarkable thermal properties. These properties are crucial for applications that require efficient heat transfer, such as in nuclear fission reactors and solar power plants.
In particular, FLiNaK molten salt, a eutectic mixture of lithium fluoride (LiF), sodium fluoride (NaF), and potassium fluoride (KF), is a promising material for reactor construction, distinguished by its high thermal conductivity.
In these contexts, FLiNaK serves as a coolant and heat carrier, with its specific heat capacity and density being crucial for system design.
In molten salts reactors, an advanced type of nuclear reactors, FLiNaK is used as a carrier medium for fissile material. These reactors utilize the high melting points and excellent heat transfer capability of molten salts to enable more efficient and safer nuclear fission.
They offer several advantages compared to conventional water-based reactors, such as higher operating temperatures enabling improved thermal efficiency, and lower operating pressures reducing the risk of steam explosions.
In thermal solar power plants, molten salts are used as a heat transfer medium to store and transport the solar energy absorbed by solar collectors. Their ability to store heat over longer periods allows for continuous power generation even when direct sunlight is unavailable.
This significantly enhances the overall efficiency and reliability of such facilities by ensuring a consistent energy supply regardless of the time of day or weather conditions.
The importance of thermochemistry and thermophysical properties for the investigation of molten salts
Thermochemistry and thermodynamics are crucial tools in the study of molten salts, providing comprehensive insights into the properties of these materials.
Gibbs energy, enthalpy, entropy, and heat capacity are parameters of great importance. Gibbs energy measures the thermodynamic potential of a system to perform work and plays a key role in determining the direction and extent of chemical reactions as well as the phase equilibria of molten salts. Furthermore, it influences the stability of these salts, which is fundamental for understanding and optimizing their applications and uses.
The thermophysical properties of molten salts are equally relevant. These properties include heat capacity, thermal conductivity, and heat transfer coefficient. They play an essential role in modeling, designing, and operating systems utilizing molten salts, such as molten salt reactors, heat exchangers, and storage tanks.
The density of molten salts affects flow properties and heat distribution, while thermal conductivity significantly influences the efficiency of heat transfer in these systems. The heat transfer coefficient is a crucial factor for the efficiency of heat exchange processes in molten salts.
In the research and development of molten salt-based technologies, a thorough investigation of thermochemical and thermophysical properties is essential. This knowledge enables the improvement of performance and efficiency of such systems, making them suitable for a wide range of applications in areas such as nuclear energy, solar energy, metal extraction, and electrochemistry.
STA, Laser Flash Thermal Diffusivity Analyser and Dilatometer are powerful tools for the analysis of molten salts
The combination of different analytical techniques enables an in-depth investigation of the thermochemical and thermophysical properties of molten salts.
Developing a comprehensive understanding of these materials is crucial to optimise their applications in various key areas.
Simultaneous Thermal Analysis (STA)
One of the techniques used is the Simultaneous Thermal Analysis (STA), a combination of Thermogravimetric Analysis (TGA) and Differential Scanning Calorimetry (DSC), which allows for the determination of various properties.
With STA, the mass loss, melting point, phase transitions, heat capacity, thermal stability, and decomposition of molten salts can be analyzed, and coupled instruments such as a mass spectrometer can also be used to examine resulting gases.
For example, STA can be used to define the thermal decomposition of lithium nitrate, an important component of some molten salts.
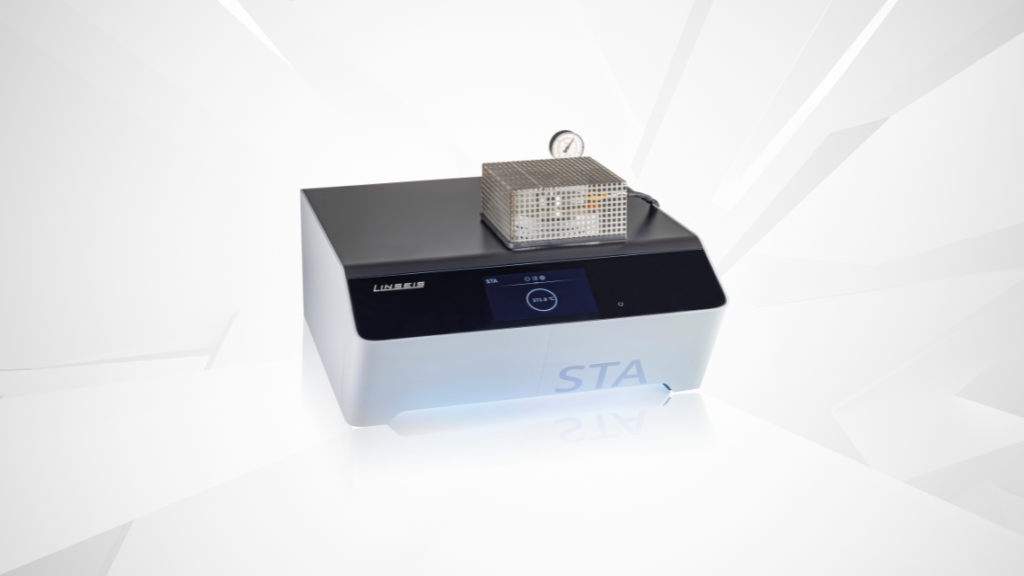
Laser Flash Analysis (LFA)
Another valuable technique is the Laser Flash Analysis (LFA), which can measure the thermal diffusivity and thermal conductivity of molten salts of various compositions at high temperatures.
For example, the thermal diffusivity of sodium chloride, a commonly used molten salt, can be measured using an LFA.
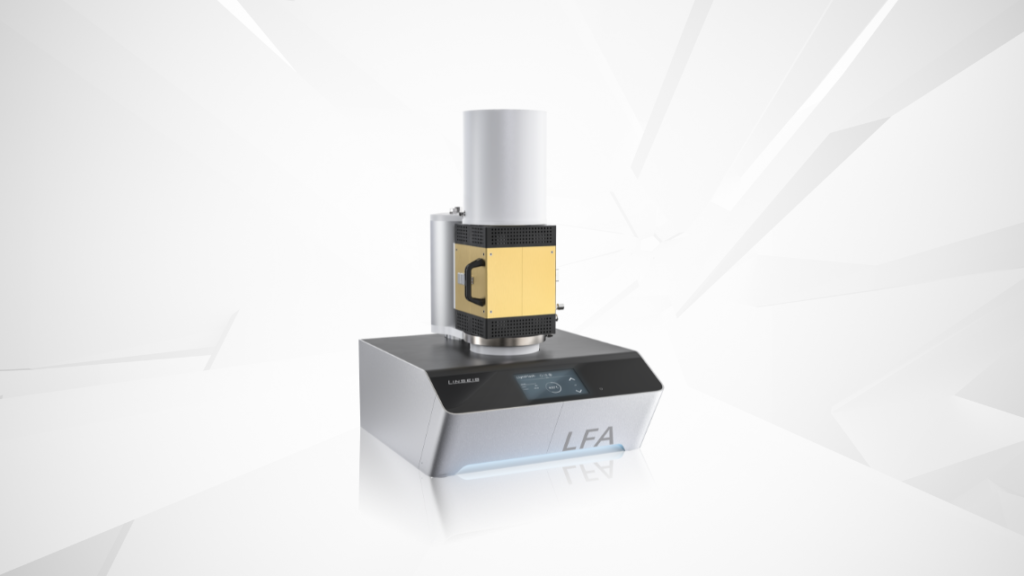
Dilatometry (DIL)
Dilatometry (DIL) is another important technique used to measure the thermal expansion of molten salts at different temperatures.
This data is particularly relevant for the design of molten salts reactors, where materials expand and contract under varying temperatures.
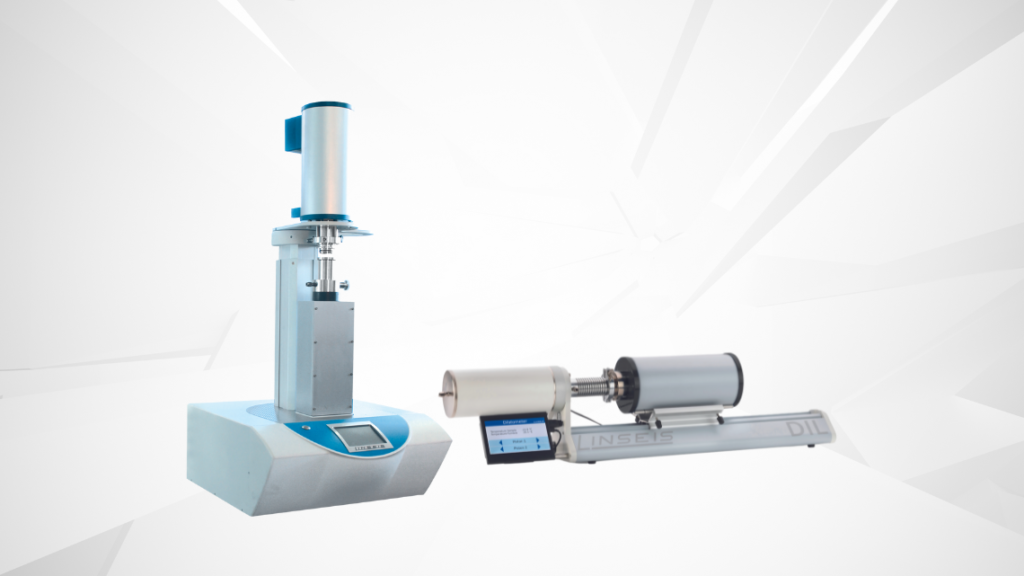
In the research and development of Molten Salts technologies, these analytical techniques are essential to characterise the material properties and understand their behaviour under different conditions.
This understanding is key to the optimisation and further development of Molten Salts applications in various industrial and scientific fields.
Simultaneous TG-DSC measurements on Molten Salts
The importance of heat storage and heat transfer is increasing significantly in the context of the growing production of alternative energy sources, such as in solar power plants where molten salts are used as heat transfer fluids and PCM (Phase Change Materials).
The efficiency of the salts used depends on material properties such as latent heat of fusion, heat capacity, density, thermal conductivity, volumetric expansion, etc.
Therefore, various methods of thermal analysis are suitable for characterizing the efficiency of molten salts.
Application Note: Stability of Molten Salts by Simultaneous Thermal Analysis (STA PT 1000)
In this study, results of TG-DSC measurements on Calcium Nitrate Tetrahydrate – Ca(NO3)2 . 4H2O – are presented and discussed. This salt is widely used as a material for heat storage and heat transfer due to its cost-effectiveness and high efficiency.
The sample was analyzed using a Linseis STA PT 1000 instrument, which simultaneously monitors the weight change and the DSC signal. From the DSC signal, the enthalpy of phase transitions and the heat capacity can be determined.
The sample was heated in a closed aluminum crucible up to 180°C at a heating rate of 10 K/min and held isothermally for 3 hours. Subsequently, it was heated to 600°C at a heating rate of 10 K/min.
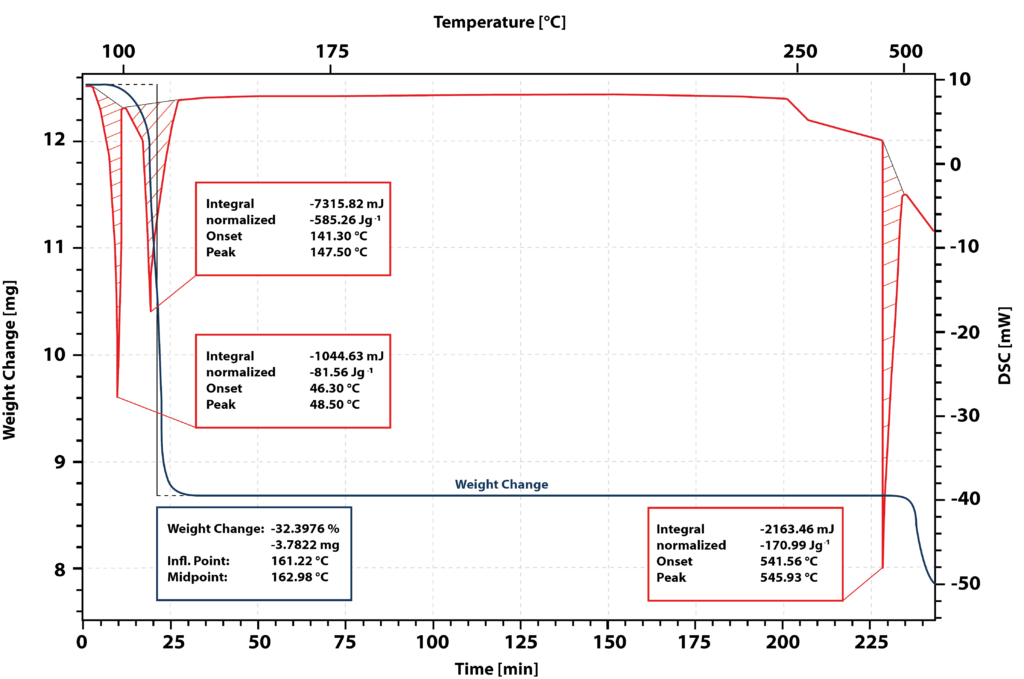
Results and discussion
Figure 1 shows the results of the measurement. The blue curve represents the mass loss, and the red curve represents the DSC signal.
The first peak in the DSC signal corresponds to the melting of the sample. The onset of the melting peak is at 46°C.
After complete melting of the sample, a second endothermic peak emerges with an onset at 141°C. The TG signal exhibits a weight loss of 32% in this temperature range, indicating the dehydration of calcium nitrate tetrahydrate to form solid anhydrous salt.
During the isothermal hold at 180°C, the sample undergoes no further changes, indicating that this temperature is ideal for drying the salt and obtaining the anhydrous salt.
Upon reheating to 541°C, an endothermic peak is observed, corresponding to the melting of the anhydrous salt. However, the TG signal shows a weight loss, suggesting decomposition of the salt upon melting. Therefore, the enthalpy of fusion and heat capacity of the molten anhydrous salt cannot be directly measured.
However, this can be achieved through further TG-DSC measurements of salt mixtures. Calcium nitrate needs to be mixed with lithium, sodium, or potassium nitrate at different mole percentages. From the DSC melting peaks of the mixtures, the enthalpies of fusion can be determined. The enthalpy of fusion of pure calcium nitrate can then be calculated by extrapolating to a mole percentage of 100% relative to calcium nitrate.
The same procedure is employed to measure the heat capacity of the molten anhydrous calcium nitrate.
Conclusion
Thermoanalytical methods are highly suitable for obtaining material properties of molten salts.
Simultaneous TG-DSC analysis provides the enthalpy of fusion and heat capacity in both solid and molten states.
The mass change signals can be used to detect processes such as the evaporation of crystalline water and the decomposition of the salt.
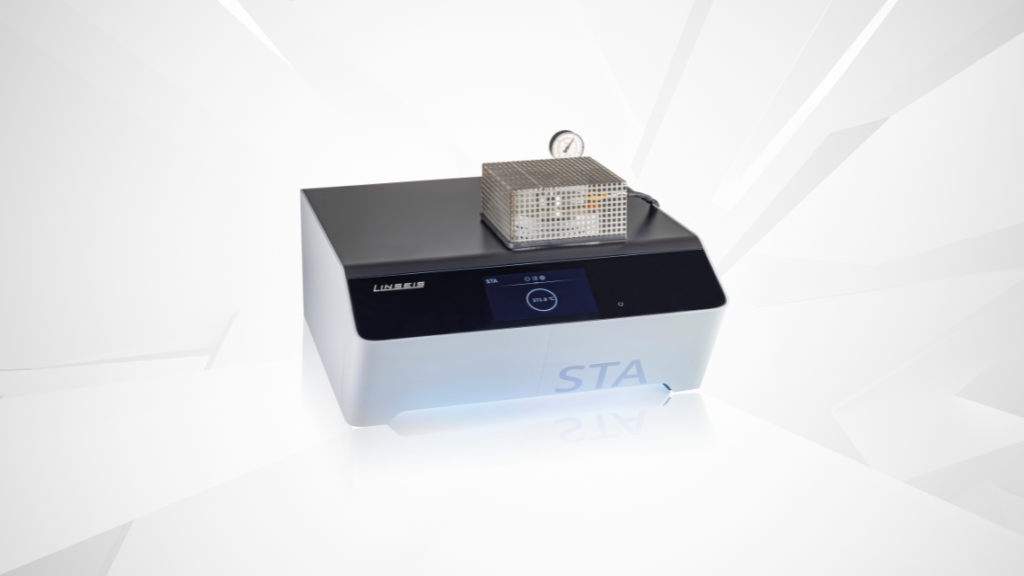
Thermal conductivity measurements on Molten Salts using the Laser Flash technique
Determining and optimizing the thermal conductivity of liquid salts is a crucial step in the development of a new generation of nuclear reactors, known as molten salt reactors or liquid salt reactors. Here, molten salts serve as both heat storage and as a medium for transferring the heat generated in the reactor core.
There are various methods for determining the thermal conductivity of liquids, each with its advantages and disadvantages. It is important to avoid generating heat losses due to convection and thermal radiation during the measurement, as these can introduce significant measurement errors and thus produce inaccurate results. Convection occurs, for example, in stationary methods due to the application of temperature gradients required for the measurement, which is exacerbated by the typically very long measurement times.
The most promising method for determining thermal conductivity in molten salts is the laser flash method because it involves an absolute measurement and thus does not require calibration with a reference material. Moreover, convection effects are minimized due to the low amount of sample required and the short measurement times.
However, since the laser flash method is primarily designed for homogeneous and solid materials, the construction of a special sample holder is required.
Figure 2 illustrates the design of the constructed sample holder. The holder is made of graphite, as it can withstand the corrosive properties of the salt even at higher temperatures. The bottom and top are attached in a way that defines the sample thickness in the middle part of the holder at a certain distance. The design also provides additional space on the sides for the material to expand at higher temperatures. Additionally, the top is equipped with holes to allow any gases generated by the material to escape. This is crucial, as dissolved gases can form bubbles leading to inhomogeneities in the material or poor contact with the holder.
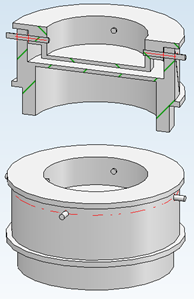
Application note: Thermal diffusivity measurement on Molten Salts using LFA 1000
The measurement of the thermal diffusivity of the molten salt FLiNaK presented here was conducted in a helium atmosphere from 773 K to 973 K using a Linseis LFA1000 system. The specially designed crucible was placed in a sample robot capable of accommodating up to three samples simultaneously. Prior to the actual test, the sample was preheated several times slightly above the melting temperature to allow degassing of the material and thus avoid bubbles in the molten salt.
Results and discussion
The thermal conductivity of the molten salt can be calculated with the aid of the thermal diffusivity measured by the LFA and the data on specific heat capacity and density using the following relationship:
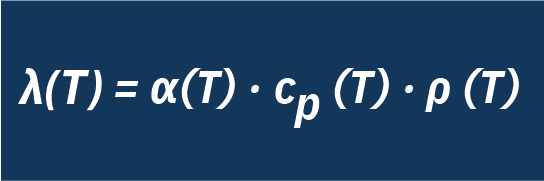
The results for thermal diffusivity and thermal conductivity are depicted in the graph below. Both properties show a relatively linear increase in values with temperature.
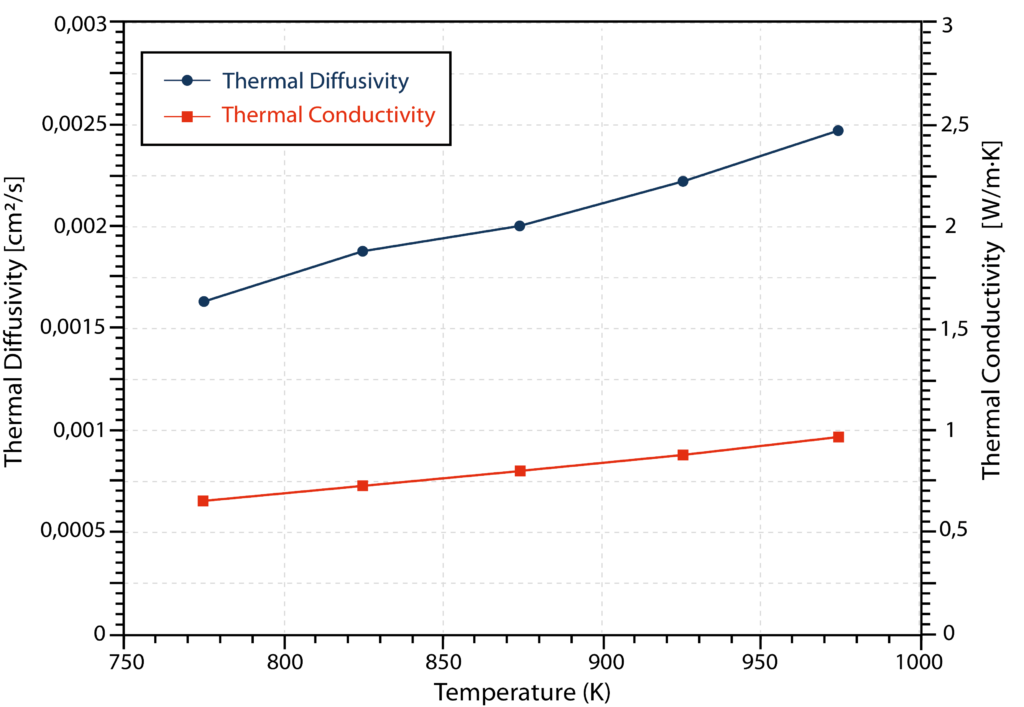
In summary, the thermal conductivity of FLiNaK molten salt was determined to be 0.652-0.927 W/m∙K with an uncertainty of +/- 0.023 W/m∙K in the temperature range of 773 K to 973 K [1]. This shows good agreement with previously published values.
Results and discussion
In conclusion, the Laser Flash technique, combined with the specially developed crucible and the combined model by Dusza, proves to be a reliable method for determining the thermal diffusivity of molten salts at high temperatures.*