Table of Contents
Introduction and Basics of Heat Spreaders
Classification and Applications of Heat Spreaders
Heat spreaders can be classified as:
- Metallic Heat Spreaders
- Phase-Change Devices
- Thermal Transfer Compunds
- Heat Pipes
- Fans
- Enclosure
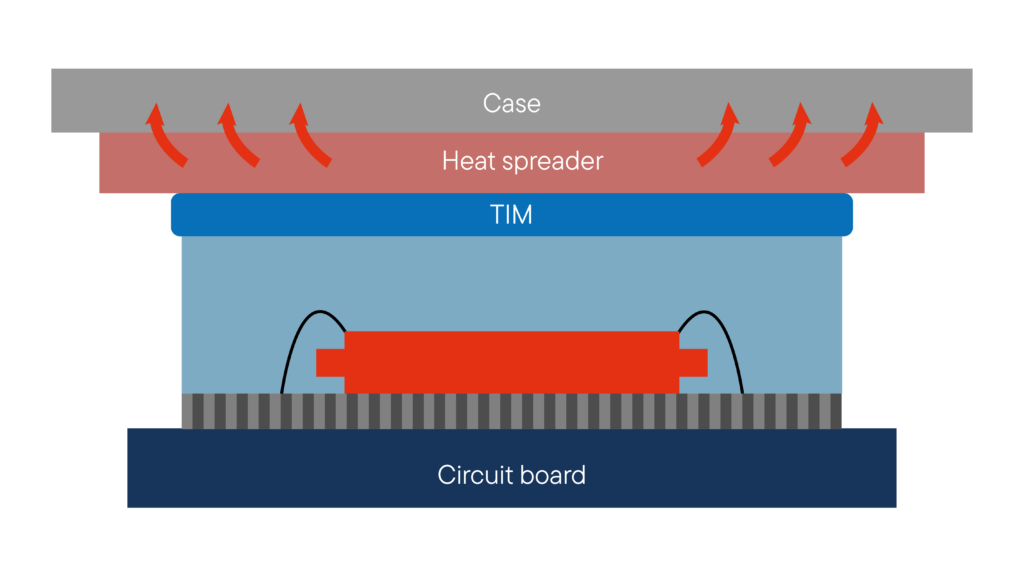
They are utilized in a variety of applications, including computer processors, mobile devices, and automotive electronics.
High thermal conductivity, low thermal resistance, large surface area, heat sink design, and thermal interface materials (TIM) are some of the properties of heat spreaders as shown in fig.1.
Heat spreaders have the ability to prevent component damage, improve performance, and dissipate heat away from electronic components. Electronic devices in various applications can benefit from the use of heat spreaders to manage thermal issues and enhance their performance and longevity.
Heat spreaders are commonly used for:
- Air-cooling circuits with integrated components, such as microprocessors.
- Cooling photovoltaic cells in concentrated photovoltaic systems
- Submounts for high-power integrated circuits and laser diodes
- Aerospace and satellite equipment, such as high-power radio-frequency devices
- LED lighting systems
- High-performance CPUs and GPUs in computers and servers
- High-power laser diodes and laser optics
- Power electronic components
- Electric vehicles need to manage battery system temperature.
These applications demonstrate the versatility and importance of heat spreaders in various industries where effective heat dissipation is essential for optimum device performance and reliability.
Measurement Methods and Equipment for Heat Spreaders
The methods of thermal measurement for TIM’s can be generally divided into steadystate and nonsteady-state (transient) methods.
Thermal conductivity measurement can be done using the laser flash method and the hot-wire method are nonsteady-state measurement time-domain thermoreflectance method (TDTR) advanced TIM’s are steady-state measurements.
SteadyState and transient methods or pulsed laser heaters, which are advanced techniques used to determine the thermal conductivity of materials, including heat spreaders in ceramics.
These methods have a complete explanation of their functioning.
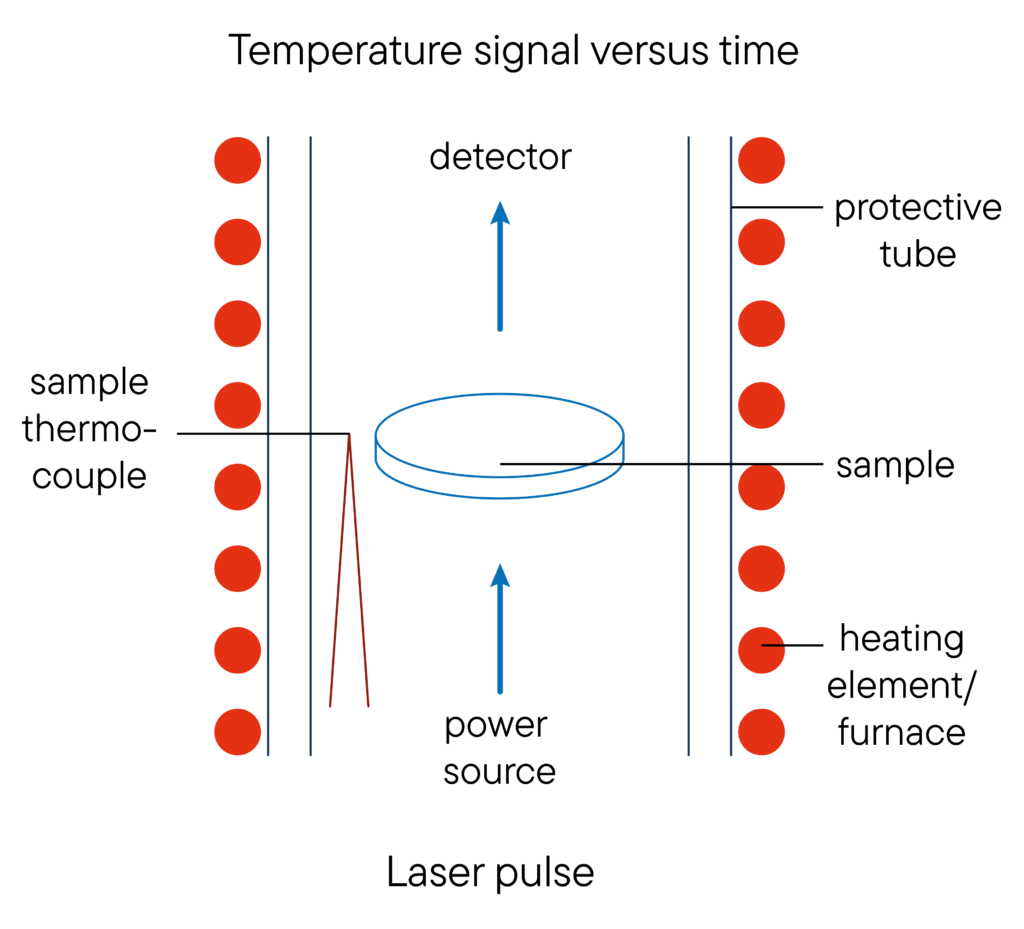
LFA (Light/Laser Flash Analyzer), TFA (Thin Film Analyzer), TF-LFA (Thin Film Laser Flash Analyzer), Periodic Laser Heating, and TIM-Tester are all measuring tools used for characterizing the thermal properties of materials, including heat spreaders.
These instruments are designed to measure the thermal diffusivity and conductivity of various materials, which are crucial for understanding heat transfer and thermal management in electronic devices and other applications.
1. LFA (Light/Laser Flash Analyzer):
The LFA (cross-plane) method is based on the principle of heating one side of a plane-parallel sample with a short pulse of energy (laser or light) and measuring the temperature increase on the opposite side using an infrared detector as represented in fig.2.
The thermal diffusivity is calculated from the time-dependent temperature rise, and the thermal conductivity is determined using the formula involving thermal diffusivity, specific heat capacity, and mass density. To calculate the thermal conductivity of the sample, the thermal diffusivity, specific heat capacity (Cp), and mass density (ρ) are used.
The formula for thermal conductivity is:
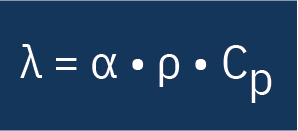
where λ is the thermal conductivity, α is the thermal diffusivity, ρ is the mass density, and Cp is the specific heat capacity.
LFA can be used for a wide range of materials, and it is based on national and international standards such as ASTM E1461, DIN EN 821, DIN 30905, ISO 8301, BS EN 1159-2, ASTM C714, ASTM C518, and more.
2. TFA (Thin Film Analyzer):
This device is designed for measuring the thermal and electrical properties of thin films. It can simultaneously measure a thin film’s thermal conductivity and in-plane electrical conductivity, as well as the Seebeck coefficient and Hall constant of a thin film sample.
3. TF-LFA (Thin Film Laser Flash Analyzer):
This is an advanced version of the standard LaserFlash, which uses a pump-probe setup (TDTR – Time-domain thermoreflectance) to characterize nm to µm thin films and coatings. TF-LFA allows the characterization of high conductive bulk materials as well.
PLH (Periodic Laser Heating):
Periodic Laser Heating uses high-energy, short-duration laser pulses to heat materials rapidly(in-plane) and selectively.
The process involves directing a laser pulse towards the sample’s surface and producing a high-energy density at the point of impact.
This energy is absorbed by the material, causing rapid heating of the surface layer above its recrystallization temperature for a specific duration before cooling.
The material’s physical and chemical properties are altered by the rapid and intense heating caused by the pulsed laser. During this process, atom migration occurs in the crystal lattice, reducing the number of dislocations and impacting the hardness and ductility of the material.
The material re-crystallizes through rapid water quenching or slow air cooling, and the heating and cooling rates affect the crystal phase composition and grain size, ultimately determining the material’s properties.
PLH are utilized in various applications, including heat spreader applications. These systems involve pulsed laser heating to achieve specific material modifications and surface treatments.
The use of pulsed lasers allows for precise control over the heating process, enabling applications like selective recrystallization of semiconductors, welding of metals, case hardening in tool steels, and phase changes in optical data storage media.
Thus, PLH plays a crucial role in various industrial and research by offering precise and controlled heating for a wide range of applications, including heat spreader applications. Additionally, the ability to perform time-resolved studies and couple with different spectroscopic techniques allows for a better understanding of the material’s behavior under high-temperature conditions, which can be useful in optimizing the performance of heat spreaders in various applications.
These techniques collectively enable precise control over the temperature distribution during pulsed laser heating for heat spreader applications, ensuring efficient and controlled heating processes while maintaining the integrity of the materials being processed.
Impact of Filler Materials on Thermal Conductivity of Heat Spreaders
The thermal resistance of a heat spreader can be greatly influenced by the filler material used.
The thermal conductivity of a heat spreader can be improved by adding filler materials to its base material, which is crucial in determining its effectiveness in dissipating heat.
The heat spreader’s thermal conductivity, thermal stability, and mechanical properties are influenced by the filler material selection
- Diamond is one of the most effective fillers for increasing thermal conductivity, with a thermal conductivity of 2000 W/m•K. The thermal conductivity of the heat spreaders can be significantly improved by its highly conductive properties.
- Boron nitride (BN)’s plate-like morphology makes it easy for particle-particle interactions and increases thermal conductivity. A composite with boron nitride can increase the thermal conductivity almost five times compared to a diamond-filled composite at a 15-volume percent loading,
- Alumina can increase thermal conductivity slightly, with an increase of 30 W/m•K. While less efficient than diamond or boron nitride, it can still improve the thermal conductivity of heat spreaders.
- Silica is commonly employed to decrease the coefficient of thermal expansion rather than improve thermal conductivity due to its poor effectiveness in increasing thermal conductivity.
- Silicone fillers are frequently utilized in thermal interface materials (TIM) to enhance heat spreading and transfer across the TIM.
Thermal Interface Materials (TIMs): Types and Applications
The Thermal Interface Material Tester, or TIM-Tester is a device that measures the thermal impedance of sample materials and determines their apparent thermal conductivity (in-plane & cross-plane).
Thermal Interface materials with a thermal conductivity of only 0.0263 W/MK, air is the worst heat conductor ever. Air inclusions between components should therefore be avoided in order to prevent heat accumulation.
Here TIM’s help to close gaps caused by unevenness, tolerances or roughness and ensure that no air gaps occur. The thermal intermediate materials are available in various designs, e.g. as:
- Heat conducting pastes,
- Heat conductive adhesive,
- Graphite and aluminium foils,
- Foam and GEL films,
- One-sided and double-sided adhesive heat conducting foils,
- Phase Change Materials (PCM’s),
- Silicone-containing and silicone-free elastomers,
- Kapton and mica discs,
- Aluminium oxide materials
In many cases it is not easy to find the right intermediate material. However, a sufficiently well-designed thermal management system is indispensable for optimum function and a long service life of electronic components.
Gap Filler
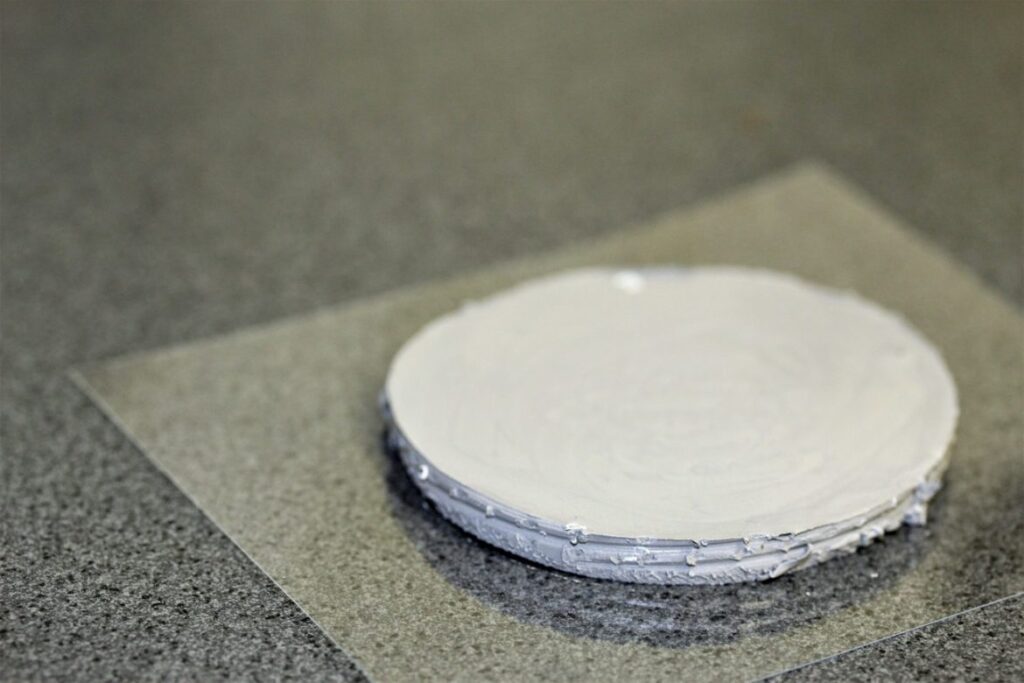
Paste
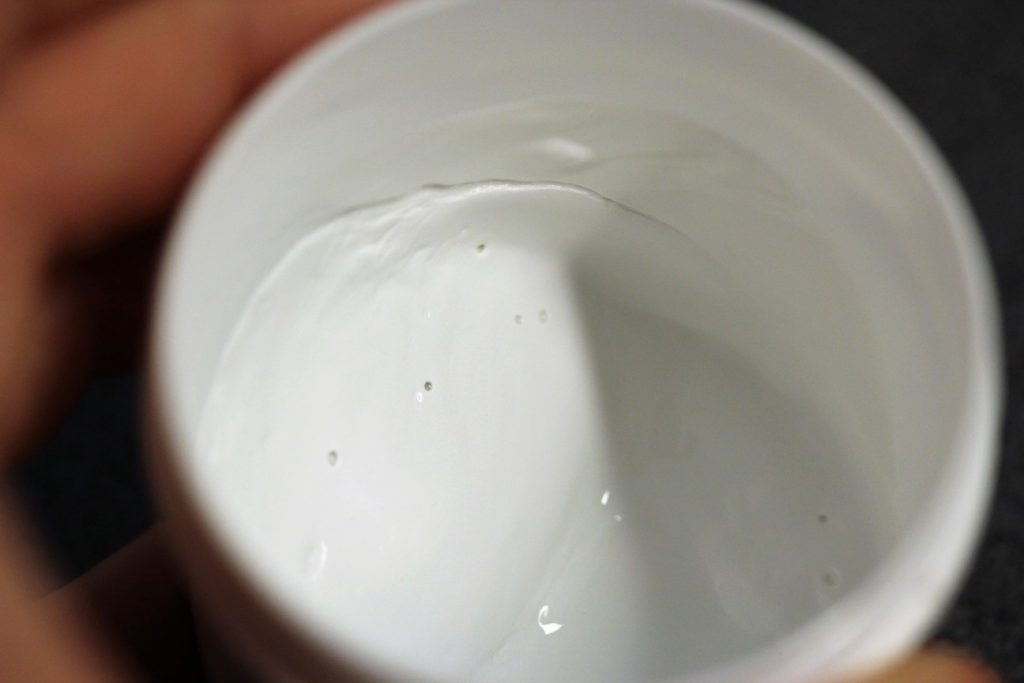
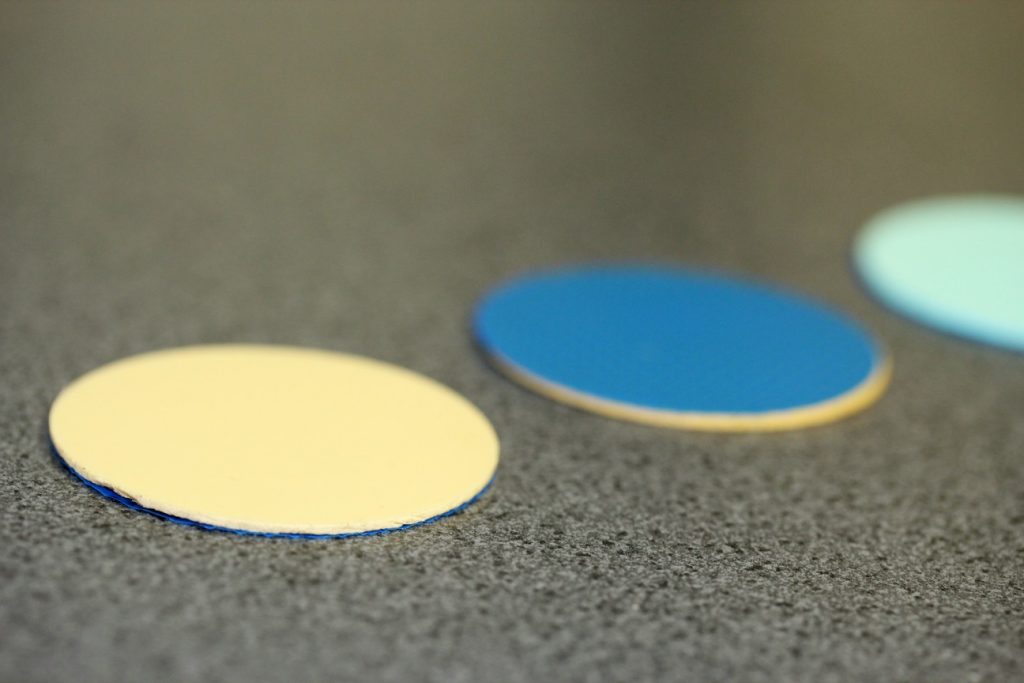
Pads
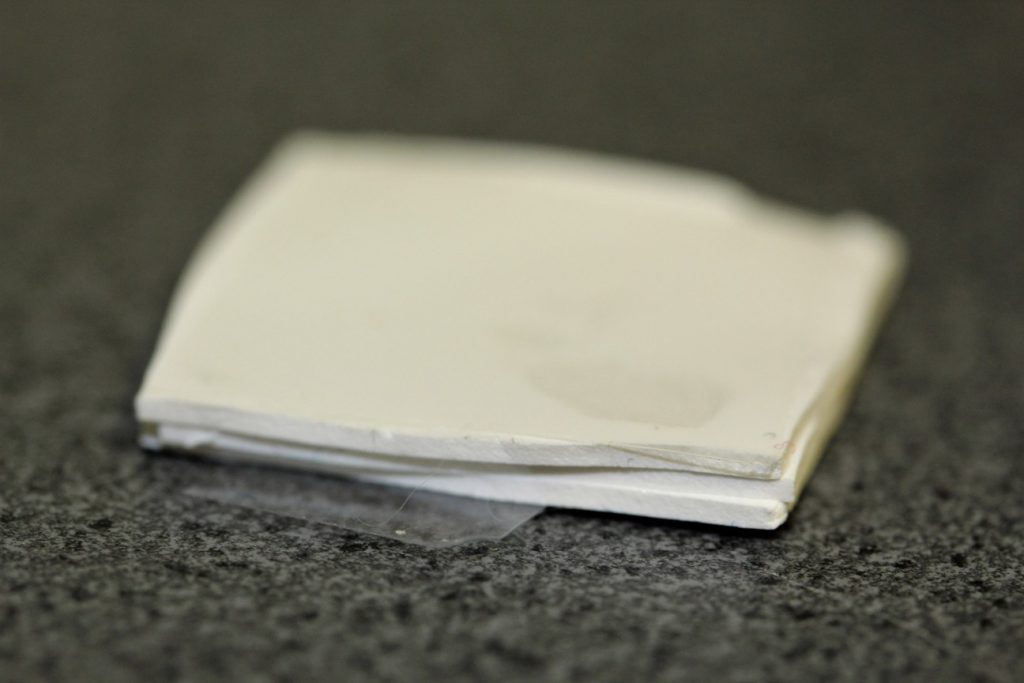
Pads as Stack
The TIM-Tester is capable of handling a variety of sample sizes and shapes, including solids, pastes, pads, and more. Operates in accordance with ASTM D5470, a standard that measures thermal resistance and conductivity of thermally conductive materials.
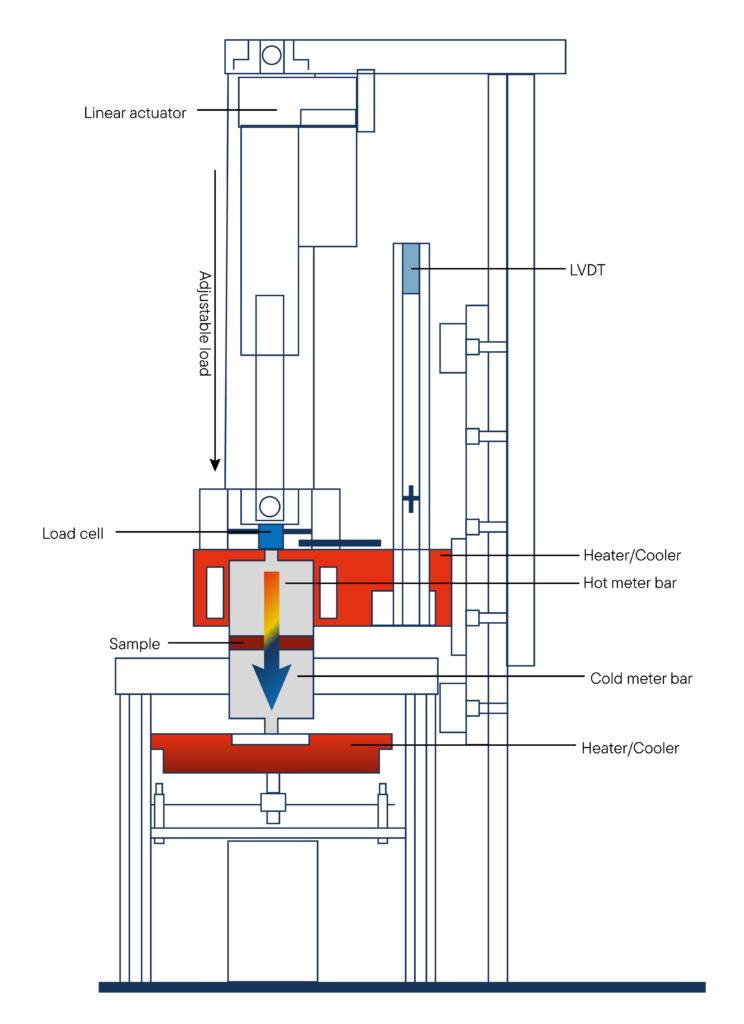
Which TIM’s are best suited for which application?
Not every material is suitable as a universal material for all applications in electronics. In order to find the perfect TIM, developers in the field of materials research must consider a wide variety of material properties such as thermal resistance, thermal conductivity, thermal impedance, mechanical tolerances of the contact pairing, temperature range, environmental compatibility and many others.
Which material is most suitable depends on the application. The three main types of TIM’s are thermal conductive films, thermal conductive pastes and thermal conductive adhesives. These differ, among other things, in their use, layer thickness, electrical insulation and thermal conductivity.
Heat conducting phases
Heat conducting pastes are often used to generate heat transfer layers, for example between a heat sink and an electronic component. These are usually applied in very small layer thicknesses of maximum approx. 50 µm. Larger component distances cannot thus be bridged. In practice, excessive amounts of paste are often used. However, too sparing application is often more critical as it may not compensate for all air inclusions.
Phase Change Materials
Phase change materials are a further development of conventional thermal pastes. As plate material these TIM’s have a continuous layer thickness, which allows a clean direct mounting on the heat sink. In addition, PCMs are characterized by their phase change temperature.
At a temperature of 45 to 55 °C, the consistency of these materials changes from solid to soft. As a result, they flow into all spaces between the components to which they are applied. If the temperature falls below the phase change temperature again, the respective medium returns to its initial state without the connection to the contact points breaking off.
Surface finish and selection of a TIM
In order to be able to use thermal pastes or adhesives, the surfaces should be almost ideal in terms of tolerance. If this cannot be guaranteed or if the handling of these materials is too complicated, films are usually used.
This allows air gaps of up to 5 millimeters to be compensated. However, the thermal resistance of these TIM’s is higher due to their greater strength.
Fields of application of thermal interface materials
The multitude of thermal interface materials produced in a wide variety of processes illustrates a change in best design practice. This change also benefits the often-desired miniaturization of components.
A higher component density reduces the air volume available for cooling and at the same time prevents the remaining air from circulating. Therefore, in systems where cooling ventilators were originally used for forced air cooling, a fanless design is usually preferred today.
TIM’s in everyday life
TIM’s are now used in a wide variety of applications, for example in automotive electronics, in the computer, memory and gaming sector, in optoelectronics and in the aerospace industry. In addition, they enable excellent heat management in electronics packaging, household appliances, lighting technology, medical technology and industrial automation.
Optimization and Industrial Applications of Heat Spreaders
Heat spreaders, which are materials that distribute heat evenly across a surface, can be measured with the TIM Tester. The TIM Tester works by applying a pressure to a sample placed between a hot and a cold meter bar.
Temperature sensors are used to measure the heat flux through the sample, and the thermal impedance can be determined by calculating the temperature drop caused by the sample material based on its geometry. The thickness of the sample can be entered manually or measured using an integrated LVDT.
High-precision measurements are the basis for optimized heat management
Due to the countless possible fields of application and the immense variety of materials, Thermal Interface Materials pose great challenges for materials research. Thermal management in the field of electronics is extremely complex and requires precise knowledge of the material properties of the applied TIMs.
In addition to measuring heat spreaders, the TIM Tester can also be utilized to test other thermal interface materials, such as thermal fluids, thermal pastes (greases), phase change materials (PCM), solders, or sturdy thermal conductors. In summary, the TIM Tester is a versatile and essential tool for measuring the thermal properties of heat spreaders and other thermal interface materials used in electronic devices that require heat spreading /shielding.
The optimization of heat spreader designs in electronics, aerospace, and other industries where efficient thermal management is essential is made possible by these methods in research and development. Thermal conductivity can be measured accurately by manufacturers to enhance heat dissipation capabilities, enhance product performance, and ensure reliability in various applications.
With this knowledge it is possible to perfect the cooperation of components and interface materials and to develop an optimal thermal management for complex electronic applications.